The moon could have formed from a seething, spinning cloud of vaporized rock, which scientists call a ‘synestia’. Scientists at the University of California, Davis and Harvard University claim that this novel lunar formation model ties several loose ends that competing and more established formation theories could not resolve.
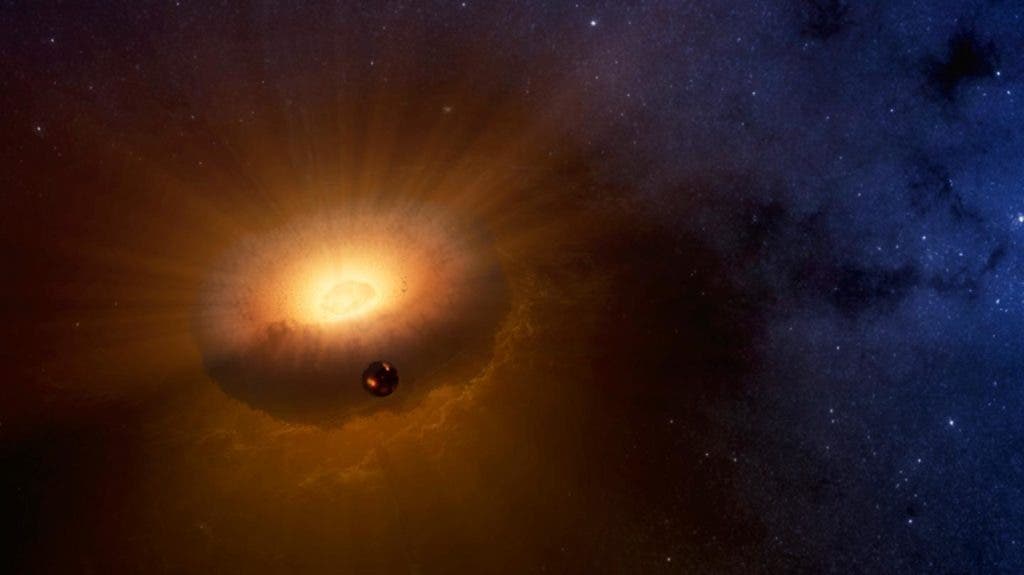
According to the mainstream lunar formation theory, early in our planet history, Earth collided with a Mars-sized object called Theia. The highly energetic collision threw molten rock and metal into orbit which eventually coalesced, forming the moon. For more than 20 years, this has been the canonical model for the formation of the moon and modern planet Earth. But, compelling as it may be, this model is likely wrong, according to Simon Lock, a graduate student at Harvard’s Department of Earth and Planetary Sciences.
Modern analyses show that both the Earth and the moon have nearly the same isotopic “fingerprint”, suggesting they both formed from an identical source. What’s more, trajectory models suggest that there’s a very narrow range of collisions that might be able to get enough mass into orbit. “There’s only a couple of degree window of impact angles and a very narrow range of sizes…and even then some impacts still don’t work,” Lock said in a statement.
“Our model explains, for the first time, how the Moon acquired its distinct composition. The Moon has a very similar composition to Earth in the major rock-forming elements (e.g., Al, Mg, Si) but depleted in elements that are more easily vaporized. Because the Moon formed within the synestia, surrounded by hot vapor, it inherited its composition from Earth but only retained the elements that are more difficult to vaporize. The more volatile elements remained in the vapor of the synestia. When the synestia cooled and contracted inside the Moon’s orbit, it took all the more volatile elements with it. We have shown that the pressures and temperatures at which the Moon would have formed inside the synestia would give it a composition similar to that observed in our Moon. Ours is the first model to be able to quantitatively explain the Moon’s composition,” Lock told ZME Science.
“Our model can also help explain why Earth and the Moon appear to have formed from the same material and why our Moon is so big,” he added.
Lock, along with colleagues from Harvard and UC Davis, have devised a new model that is better able to account for these inconsistencies. It relies on a new type of planetary object called synestia — a rapidly spinning mass of molten and vaporized rock, with some matter belonging to the body in orbit around itself. The whole thing looks a lot like a giant planetary-sized donut made of vaporized rock. “Synestia” comes from “syn-,” “together” and “Hestia,” Greek goddess of architecture and structures.
The term “synestia” was coined by Lock and Sarah Stewart, who is professor of planetary science at the University of California, Davis. Both researchers are academically focused on studying what happens when planet-sized objects smash into each other. Particularly, the two are interested in what happens during the collisions of massive spinning objects.
Any rotating object has an angular momentum which needs to stay conserved. The law of conservation of angular momentum states that when no external torque acts on an object, no change of angular momentum will occur. If the net torque is zero, then angular momentum is constant or conserved.
It’s the conservation of angular momentum that can explain why an ice skater can increase the angular acceleration by bringing his or her arms and legs close to the vertical axis of rotation. Because the moment is conserved, when the skater decreases rotational inertia, the rotation rate must increase. Ultimately, the skater’s angular momentum is the same. And when two ice skaters catch ahold of each other, the angular momentum of each skater adds up — again, per the law of conservation of angular momentum, the summed angular momentum must remain the same. As a nice bit of trivia, it’s because of this law that the solar system is flat.
Now, imagine what happens when your two skaters are actually two planets that crash into each other. When Lock and Stewart modeled what happened to Earth-sized rocky planets after a collision with other large objects over a range of high temperatures and high angular momentum, they found this completely new planetary structure.
“The main challenge in this research was breaking our preconceptions about what the aftermath of giant impacts should look like. Two of the most significant ‘Eureka’ moments were that the realization that impacts were creating a very different type of planetary structure, synestias, and that the synestia was so large that the Moon would be forming inside the vapor of the synestia,” Lock told ZME Science.
Out of the Earth-synestia, chunks of molten rock which were injected into orbit following the impact formed the seed for the moon. Synestias likely don’t last long — not more than a couple hundred years — because they can’t sustain their heat for too long, and shrink rapidly, finally collapsing into a molten planet. And while the Earth-synestia gradually shrank, vaporized silicate rock rained into the proto-moon, which explains how the moon inherited its composition from Earth. At the same time, because it formed at high temperatures (4,000-6,000°F), the moon lost the easily vaporized elements which is why the moon also has a distinct composition.
The synestia model answers many long-standing questions about Earth’s and the moon’s formation, but this work is still in its infancy. Much more work must be completed to refine the model further. For instance, scientists would like to know how the vapor that formed the moon flowed and behaved in more detail.
“The study of forming Moon-formation from a synestia is still in the preliminary stages. We have shown that each of the steps to form the Moon from the synestia are feasible, but there is a lot more detailed interrogation of each of these steps yet to do. Also, by better constraining the chemical and isotopic composition of Earth and the Moon, we can find new tests of our model to see whether it truly can produce a Moon that looks like our own,” Lock said.
“It may be possible to observe synestias in upcoming exoplanet surveys, but the lifetime of terrestrial synestias is so short and the possibility of observing one is quite low,” he added about the possibility of witnessing a synestia in the making.
“Forming the Moon within the vaporized Earth is a fundamentally new environment for Moon formation. This model changes the emphasize of the study of Moon formation from a particular impact to the properties of the synestia produced by the impact. There are a range of very different impacts that could have formed our Moon.”
If you’d like to learn more about the synestia moon formation model, Lock made a website explaining it in great detail. You’re welcome to check it out.
Scientific reference: Simon J. Lock et al. The origin of the Moon within a terrestrial synestia, Journal of Geophysical Research: Planets (2018). DOI: 10.1002/2017je005333. <