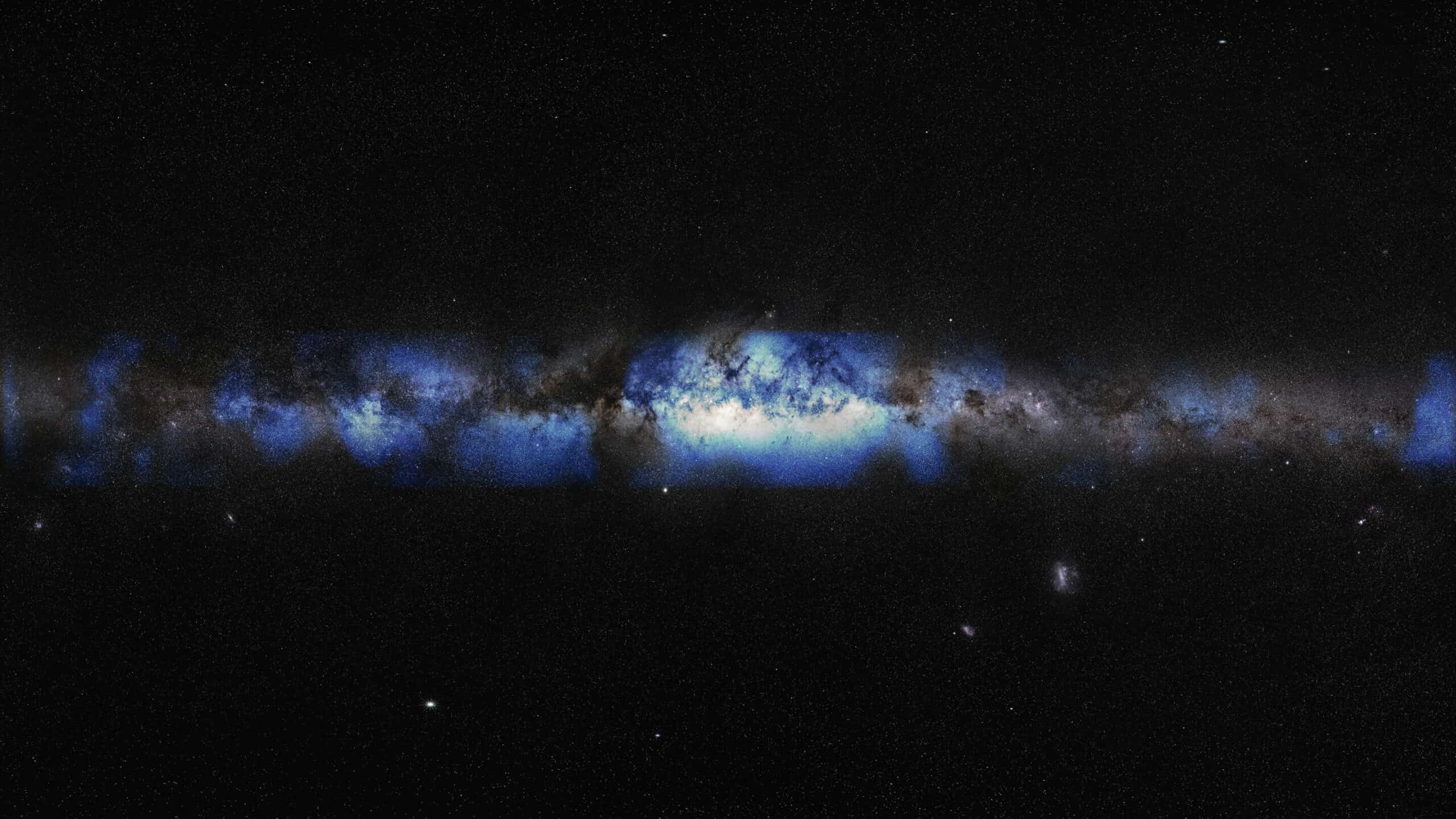
Our Milky Way galaxy, a breathtaking spectacle adorning the night sky, has always captivated our imagination. We’re all taken aback by spectacular images of our home galaxy taken by the likes of Hubble or the more recent James Webb Space Telescope. Now, scientists at the IceCube Neutrino Observatory have successfully captured the very first image of the Milky Way using the enigmatic particles known as neutrinos.
In its new study, the IceCube Collaboration, comprising an international group of over 350 scientists, presents compelling evidence of high-energy neutrino emissions originating from the core of our galactic home.
Unveiling the ghostly messengers
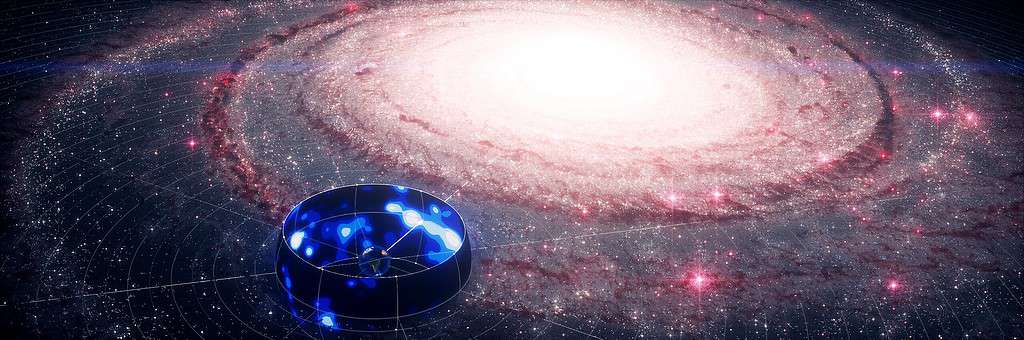
Neutrinos can be seen as the ghostlike messengers of the cosmos. They possess minuscule masses and interact only weakly with matter, including other particles and even light.
Neutrinos are fundamental particles, meaning they are not made up of smaller particles. They belong to a family called “leptons,” which also includes the familiar electron. However, neutrinos have very little mass compared to electrons, which is why they’re often referred to as “ghost particles.”
They traverse the universe unhindered, enabling scientists to explore the darkest corners of the cosmos, beyond the reach of light. Trillions of neutrinos pass through your body every second, yet you would never notice their presence because they hardly ever collide with other particles. But this also makes them incredibly difficult to detect and study.
Scientists have built specialized detectors deep underground or under large bodies of water to capture neutrinos. These detectors are designed to detect faint traces of radiation produced when a neutrino occasionally interacts with matter. By studying these interactions, scientists can learn more about the properties of neutrinos and the processes that produce them.
The IceCube detector is one such neutrino detector. Located at the South Pole, it is the largest neutrino detector in the world and operates by taking advantage of the properties of ice.
IceCube consists of thousands of spherical optical sensors, called digital optical modules (DOMs), embedded within a cubic kilometer of Antarctic ice. These DOMs are spread out over a grid, extending up to a depth of about 2.5 kilometers. Each DOM contains a photomultiplier tube that can detect the faint flashes of light produced when a neutrino interacts with the ice.
When a neutrino collides with an atomic nucleus in the ice, it produces secondary particles, including charged particles called muons and a faint bluish light called Cherenkov radiation. The Cherenkov light travels through the ice and is detected by the DOMs. By measuring the pattern and timing of these light flashes, scientists can reconstruct the direction and energy of the incoming neutrino.
IceCube is not only designed to detect neutrinos from astrophysical sources like supernovae or active galactic nuclei but also to search for high-energy neutrinos from beyond our solar system. These high-energy neutrinos are particles with energies millions to billions of times greater than those produced by stellar fusion reactions.
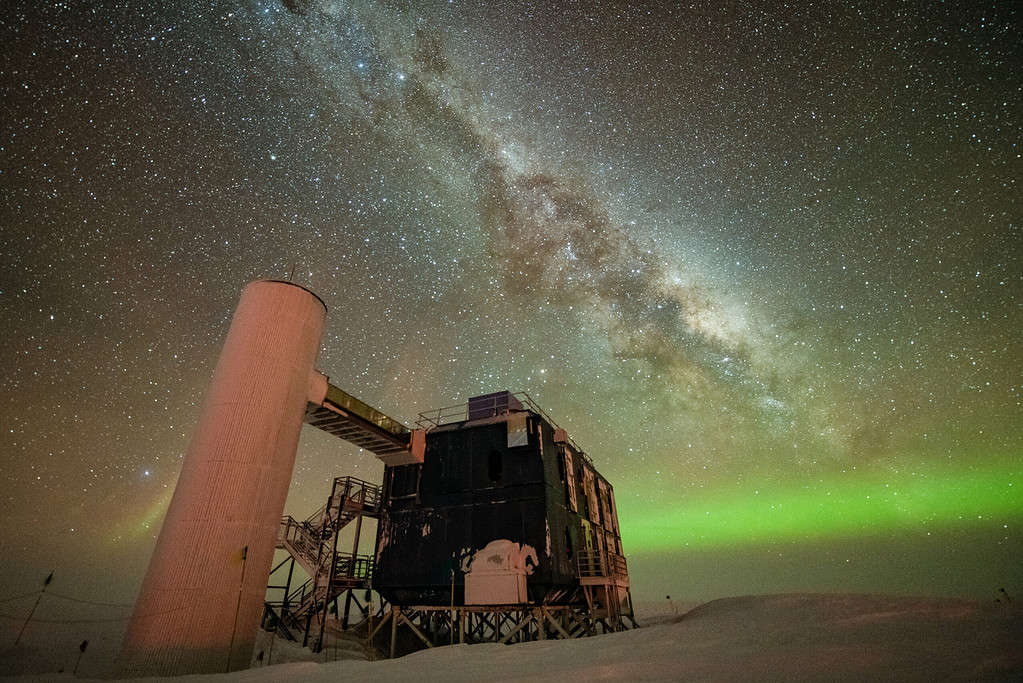
In a monumental achievement, the IceCube Neutrino Observatory has now detected high-energy neutrinos using a new technique and used these signals to piece together a new map of the Milky Way.
“What’s intriguing is that, unlike the case for light of any wavelength, in neutrinos, the universe outshines the nearby sources in our own galaxy,” says Francis Halzen, a professor of physics at the University of Wisconsin–Madison and principal investigator of IceCube.
“As is so often the case, significant breakthroughs in science are enabled by advances in technology,” says Denise Caldwell, director of NSF’s Physics Division.
“The capabilities provided by the highly sensitive IceCube detector, coupled with new data analysis tools, have given us an entirely new view of our galaxy—one that had only been hinted at before. As these capabilities continue to be refined, we can look forward to watching this picture emerge with ever-increasing resolution, potentially revealing hidden features of our galaxy never before seen by humanity.
To capture the elusive neutrinos emitted by our galaxy, the IceCube Collaboration focused their search on the southern sky, where the majority of neutrino emissions from the galactic plane are expected. However, the presence of background muons and neutrinos generated by cosmic-ray interactions with Earth’s atmosphere presented significant challenges.
To overcome these obstacles, scientists at Drexel University, collaborating with IceCube, developed sophisticated analyses targeting “cascade” events—neutrino interactions within the ice that produce spherical showers of light. By isolating these events, the contamination from atmospheric muons and neutrinos was significantly reduced, leading to a higher purity of astrophysical neutrinos originating from the southern sky.
Empowering astronomy with AI
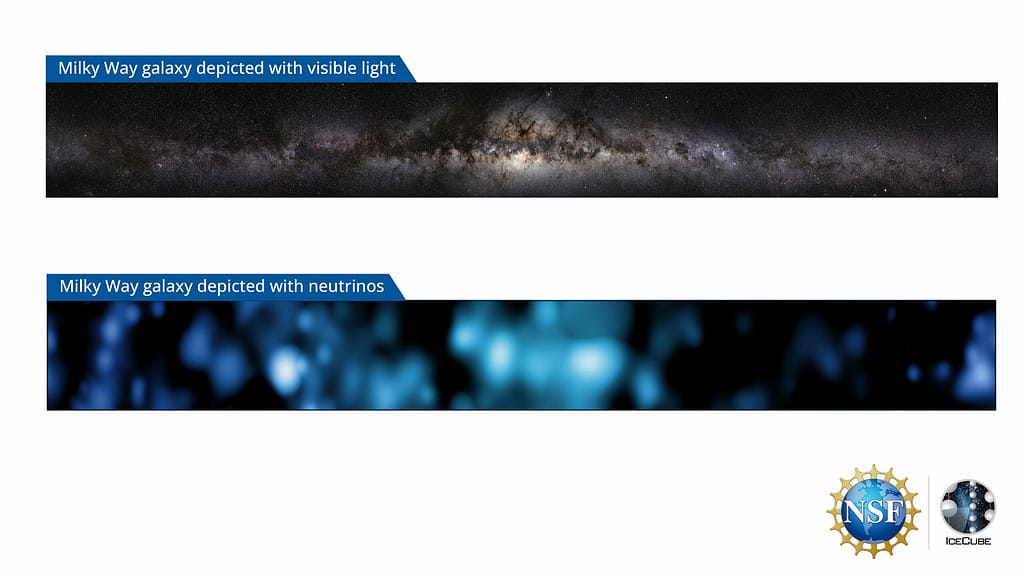
The true breakthrough in this endeavor came with the integration of machine learning techniques developed by researchers at TU Dortmund University, also part of the IceCube Collaboration. These advanced methods further enhanced the identification of neutrino-induced cascades, enhancing the accuracy of direction and energy reconstruction.
Mirco Hünnefeld, a physics Ph.D. student at TU Dortmund University and co-lead analyzer, highlights the impact of these advancements: “The improved methods allowed us to retain over an order of magnitude more neutrino events with better angular reconstruction, resulting in an analysis that is three times more sensitive than the previous search.”
Cosmic rays, consisting of high-energy protons and heavier nuclei, also originate from within our galaxy. As they interact with galactic gas and dust, they give rise to gamma rays and neutrinos. The detection of gamma rays emanating from the galactic plane had already indicated the potential for the Milky Way to be a source of high-energy neutrinos.
The dataset employed in this study encompassed an astonishing 60,000 neutrinos spanning a decade of IceCube data. This vast collection is thirty times larger than the selection used in previous analyses of the galactic plane based on cascade events. These neutrinos were meticulously compared to prediction maps derived from gamma-ray observations of the Milky Way made by the Fermi Large Area Telescope, as well as alternative maps formulated by a group of theorists known as KRA-gamma.
“The strong evidence for the Milky Way as a source of high-energy neutrinos has survived rigorous tests by the collaboration,” said Ignacio Taboada, a physics professor at the Georgia Institute of Technology and IceCube spokesperson.
With this remarkable milestone achieved, the next step lies in identifying specific sources within our galaxy. IceCube’s planned follow-up analyses aim to tackle these intriguing questions and unlock the secrets of the Milky Way, allowing us to witness the universe through an entirely new lens.
“Observing our own galaxy for the first time using particles instead of light is a huge step. As neutrino astronomy evolves, we will get a new lens with which to observe the universe,” said Naoko Kurahashi Neilson, a physics professor at Drexel University and member of IceCube.
The findings were reported in two studies published in the journal Science at the same time (first and second).