
The mystery of how complex carbon molecules with a ‘soccer-ball’ type structure–nicknamed buckyballs–came to be found in interstellar space has puzzled scientists for some time.
But now, a team of researchers from the University of Arizona have proposed a potential formation mechanism for carbon-60 (C60)–a spherical molecule comprised of 60 carbon atoms in ring-like structures–in space.
The team discovered that silicon carbide dust left behind by dying stars then bombarded by high energy particles and extreme temperatures could shed silicates leaving behind pure carbon needed to create C60.
Their results are published in the journal Astrophysical Journal Letters.
The detection of buckyballs–named for their similarity to the dome-like architecture of Buckminister Fuller– and even larger C70 molecules a few years ago caused a rethink of the theory that such molecules could only be formed in the lab.
Additionally, and more importantly, the discovery overturned the idea that only light molecules–up to around 10 atoms–could be found scattered through interstellar space.
Another surprise emerged from the fact that the molecules detected were pure carbon.
In the lab, C60 is created by blasting together pure carbons sources such as graphite. This process should be almost impossible in the planetary nebulae that the interstellar C60 was found. This is because this environment– debris created in the violent death throes of stars–has about 10,000 hydrogen molecules for every carbon molecule.
Any hydrogen should destroy fullerene synthesis,” says Jacob Bernal, an astrobiology and chemistry doctoral student and lead author of the paper. “If you have a box of balls, and for every 10,000 hydrogen balls you have one carbon, and you keep shaking them, how likely is it that you get 60 carbons to stick together?
“It’s very unlikely.”
Bernal and his team began investigating this conundrum with the aim of uncovering a potential C60 formation mechanism when they realised that the transmission electron microscope (TEM) located at the Kuiper Materials Imaging and Characterization Facility at the University of Arizona, was able to simulate the planetary nebula environment fairly well.
TEM’s 200,000-volt electron beam is able to probe matter down to 78 picometers in order to see individual atoms. The beam also operates in a vacuum with extremely low pressures. The incredibly low-pressure in TEM is very close to the pressure found in circumstellar environments. But this is more by luck than design.
“It’s not that we necessarily tailored the instrument to have these specific kinds of pressures,” explains study co-author Tom Zega, an associate professor in the Univerity of Arizona Lunar and Planetary Lab. “These instruments operate at those kinds of very low pressures not because we want them to be like stars, but because molecules of the atmosphere get in the way when you’re trying to do high-resolution imaging with electron microscopes.”
The team drafted the assistance of the U.S. Department of Energy’s Argonne National Lab, Chicago, which has a TEM capable of studying the radiation responses of materials. Placing silicon carbide–a common form of dust produced by stars– in the low-pressure environment of the TEM, the team in Chicago subjected it to temperatures up to 1,830 degrees Fahrenheit whilst bombarding it with high-energy xenon ions.
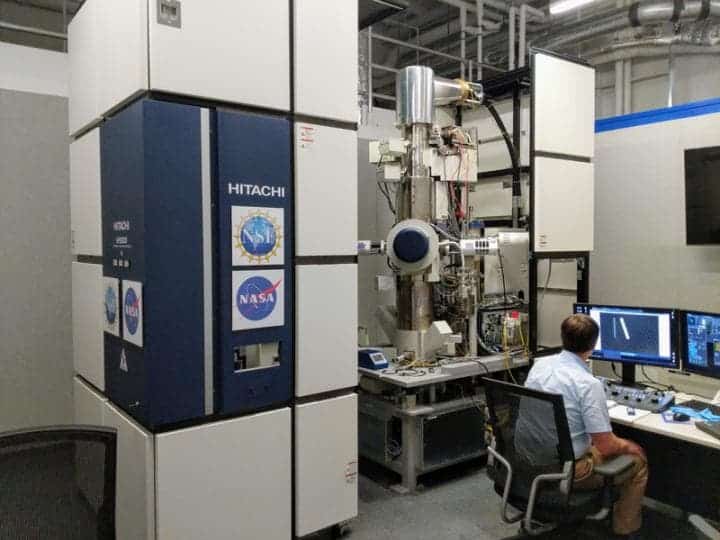
Following this, the sample was returned to the University of Arizona so researchers could employ the higher resolution and better analytical capabilities of the TEM located there. The team’s hypothesis would be validated if they observed the silicon shedding and exposing pure carbon.
Sure enough, the silicon came off, and you were left with layers of carbon in six-membered ring sets called graphite,” adds co-author Lucy Ziurys, Regents Professor of astronomy, chemistry and biochemistry. “And then when the grains had an uneven surface, five-membered and six-membered rings formed and made spherical structures matching the diameter of C60.
“So, we think we’re seeing C60.”
This work suggests that C60 is derived from the silicon carbide dust made by dying stars–hit by high temperatures, shockwaves and high energy particles. These violent conditions leech silicon from the surface and leaving carbon behind.
These big molecules are dispersed because dying stars eject their material into the interstellar medium – the spaces in between stars – thus accounting for their presence outside of planetary nebulae.
Buckyballs are very stable to radiation, allowing them to survive for billions of years if shielded from the harsh environment of space.
“The conditions in the universe where we would expect complex things to be destroyed are actually the conditions that create them,” says Bernal, also adding that the implications of the findings are endless.
“If this mechanism is forming C60, it’s probably forming all kinds of carbon nanostructures,” Ziurys concludes. “And if you read the chemical literature, these are all thought to be synthetic materials only made in the lab, and yet, interstellar space seems to be making them naturally.”
Original research: “Formation of Interstellar C60 from Silicon Carbide Circumstellar Grains,” The Astrophysical Journal Letters, 2019.