On April 22, 2022, deep beneath the Franco-Swiss border near Geneva, Switzerland, two beams of protons were accelerated around a 27-kilometre ring colliding and creating a shower of secondary particles. The experiment is nothing new for CERN’s Large Hadron Collider (LHC). In fact, colliding at an injection of 450 billion electronvolts (450 GeV) the experiment is well below the power that this, humanity’s largest and most powerful particle accelerator can achieve.
Yet, it is what this modest test of the LHC represents that has physicists excited. The test marks the beginning of a new series of experiments with the LHC which will collide particles up to an energy of 13.6 trillion electronvolts (TeV), the most powerful collisions at the accelerator to date. And this is just the beginning of what should be an exciting new period for particle physics.
This third experimental period of the LHC–known as Run 3–will lead to another extended break in 2026. During a three-year pause lasting until 2029, the LHC will undergo its most substantial transformation yet —completing the high luminosity upgrade which began in 2018. Following this, the luminosity of the LHC will be increased by an estimated factor of 10.

The luminosity of the LHC refers to the number of particles it is able to collide, and an increase in collisions means an increased chance to spot exotic, hitherto unseen physics. This means the resultant accelerator, the High Luminosity Large Hadron Collider (HL-LHC) will have the power to probe the physics that governs the universe beyond what is known as the Standard Model of Particle Physics.
Beyond the Standard Model
It’s only fitting that it should be the LHC that humanity turns to in order to search for physics beyond the Standard Model–the best description we have of the particles and the interactions that govern the subatomic world. It was, after all, with this tremendous piece of apparatus that this model, first devised in 1971, was completed.
In July 2012 in a lecture hall at CERN in Switzerland the discovery of the Higgs boson, detected by the LHC ATLAS and CMS experiments, was announced. The boson– a force-carrying particle– represented the last particle to be predicted by the Standard Model. Thus its discovery, which would scoop the Nobel Prize in Physics in 2013, represented the completion of that model.
Further to this, as the mediating particle of the so-called Higgs field, the Higgs boson is the particle that is responsible for granting most other inhabitants of the particle zoo their mass. That means that its discovery also marked the long-standing issue in physics of how most particles get their mass.
Yet, despite the sense of finality, that statement may suggest, this was by no means the final element of physics to be discovered. There are elements of physics not described by the Standard Model, like the nature of dark matter and what gives neutrinos their tiny almost insignificant mass.
Likewise, there are still questions remaining regarding the Higgs boson itself, which isn’t exactly the particle that was predicted to exist before its discovery.
It’s these questions and lingering puzzles that the LHC is now in a position to begin probing.
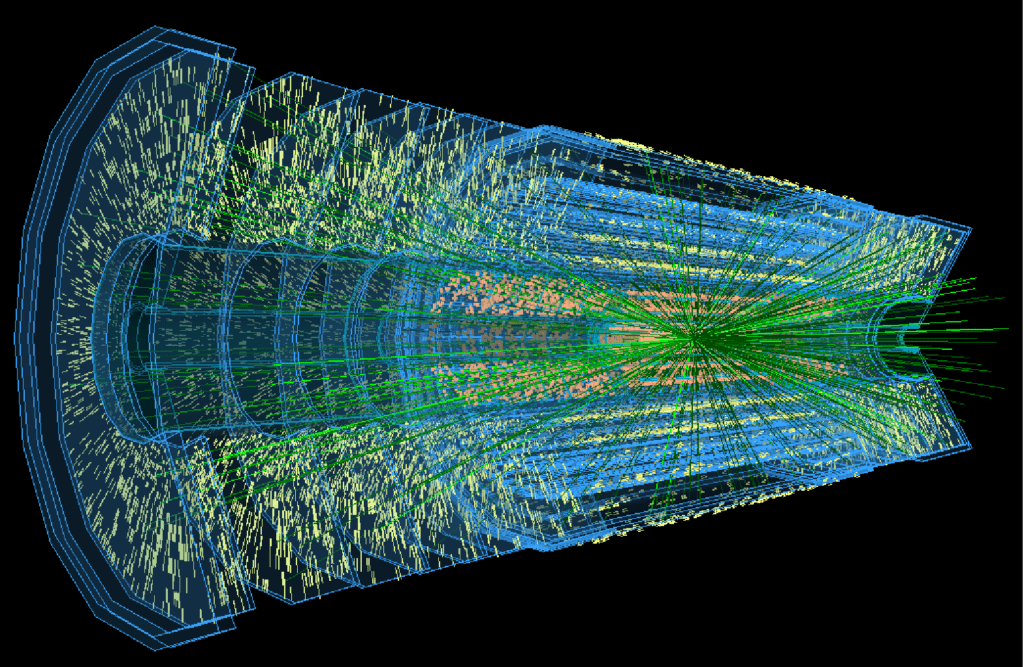
“We have discovered how particles like the electron acquire mass via interactions with the Higgs boson, completing the ‘standard model’ – the most successful theory of nature known to humans. However, there are many observations that are not predicted by this model,” says Salvatore Rappoccio of the University of Buffalo, New York, USA, who searches for new physics using the Compact Muon Solenoid (CMS) experiment located at the LHC.
Rappoccio told Elsevier: “After the discovery of the Higgs boson, new physical interactions have not been observed at the LHC. This leads us to the conclusion that, if they exist, they are either at energies higher than the LHC’s capability [of around 13 TeV] or have extremely low production probabilities in our collisions and are hidden among background processes.”
One of the questions Rappoccio and his team will be looking to answer is why is the Higgs Boson discovered at the LHC slightly different from the particle that is predicted by the Standard Model.
A better understanding of the Higgs boson and the physics surrounding it could be delivered by the fact that the HL-LHC will be capable of creating far more of the particles than the LHC was capable of. In 2017 the LHC created around 3 million Higgs particles. Operators at CERN estimate that in 2029, the HL-LHC will create an estimated 15 million Higgs bosons.
But, the HL-LHC won’t just be able to probe the laws of physics in the universe as it exists today. Perhaps, even more impressively, the HI-LHC will be capable of replicating the conditions found immediately after the Big Bang, thus giving us the clearest picture of our infant universe ever.
The Large Hadron Collider: Travelling Back to the Dawn of the Universe
The LHC doesn’t just smash together beams of protons and nor will its successor, the HL-LHC. The world’s largest particle accelerator is also capable of smashing together much heavier particles–even atoms of the element iron stripped of electrons.
The collision of iron ions is far less common at the LHC than proton-proton collisions, with one month a year devoted to this kind of experiment, but that isn’t to say it hasn’t been fruitful. In 2020, researchers at CERN were able to create quark-gluon plasma, a state of matter significant because it existed in the earliest moments of the universe, shortly after the Big Bang.
In the extreme conditions created at the LHC, protons, and neutrons that make up the lead ions “melt” in the process freeing quarks from their bonds with the gluons. Watching how quark-gluon plasma both expands and cools, gives researchers a hint as to how it gradually gave rise to the particles that make up the universe as it also cooled and expanded in its infancy.
Such studies are also essential to understanding the interactions of one of the universe’s four fundamental forces, the strong nuclear force. This discipline, known as Quantum Chromodynamics (QCD), describes the interactions between quarks and gluons.
The LHC isn’t the first machine to replicate this state of matter, but it improves on previous efforts by creating hotter, denser and longer-lived quark-gluon plasma permitting physicists to study this state of matter in unprecedented detail.
Quarks and gluons are only usually found contained within other particles like protons and neutrons. They only exist freely at incredibly high energies such as those that existed in the early universe when it was in an incredibly hot and dense state before inflation had caused it to expand and cool.

Using the LHC’s ALICE detector researchers at the accelerator have been able to estimate the temperature of the quark-gluon plasma by using photons emitted by this state of matter in addition to determining its energy density, both of which have yielded results higher than previous estimates. The scientists at CERN have also been able to use particles created by this dense hot “soup” of matter to probe its shape and other qualities.
Thanks to the upgrades of the LHC the ALICE detector, the key instrument in measuring the particles created by heavy-ion collisions, has received a massive boost.
During Run 3 CERN expects the ATLAS and CMS experiments to achieve more collisions than achieved in its other two periods of operation combined, while LHCb will have its collision count increased by three times. The effect on ALICE will be even more intense, this detector will in the future be able to measure up to 50 times as many heavy-ion collisions as before.
More collision events mean creating more quark-gluon plasma and a longer-lasting state of this primordial matter and providing researchers with more data to study the conditions of the early universe.
“The coming decade at the LHC offers many opportunities for further exploration of the quark-gluon plasma,” ALICE experiment spokesperson Luciano Musa said in a CERN press release. “The expected tenfold increase in the number of lead-lead ion collisions should both increase the precision of measurements of known probes of the medium and give us access to new probes. In addition, we plan to explore collisions between lighter nuclei, which could cast further light on the nature of the medium.”