- Stanford researchers claim the inherent disordered molecular structure of polymer semiconductors is actually desirable.
- Current organic solar cells made with semiconducting polymers try to mimic orderly silicon structures as much as possible; this direction of research may be fundamentally wrong according to the Stanford researchers.
- A balance between molecular order and disorder at the polymer semiconducting interface is required; if following work delivers significant results then it might change the face of the industry as it is today.
Organic solar cells are maybe the next best thing in renewable energy. They’re light, flexible and cheap. You could even have solar cells embedded into your clothing that would twist and flex along with your movements, while generating electricity – of course the generated power is very low, but it’s well thinking about it just so you can grasp the potential of organic solar cells. Think of entire buildings covered with these over the windows or equipped on all sorts of mobile applications where stiff solar cells just don’t cut it. You’re convinced by now, but why aren’t we seeing these everywhere? You might have guessed by now – very poor performance.
A paradigm shift
A new research from Stanford University, however, may help improve organic solar cell photon-energy conversion efficiency. Its implications are far and wide, though, since it implies a total paradigm shift from how scientists currently approach organic solar cells. Namely, instead of focusing on increasing structural molecular order in the polymer crystals, scientists should actually rejoice of the inherent disordered nature of polymers (plastics). Their work suggests that if an ideal structure that guides electrons from the disordered polymer interface to more orderly structures onward, a better performance may be reached.
Now, it’s important to understand that great strides have been made in the past few years. Just a few years ago, efficiency was around 4% while currently 12% and onward efficiency can be seen – for comparison current commercial single junction solid silicon solar cells have a rated efficiency of around 25%; in the lab, the current record for solar energy conversion is 44.7% with multi-junction cells and concentrated solar power. Current efforts in harnessing solar energy using organic solar cells are being hampered, however, by the lack of an exhaustive and fundamental understanding of how these convert the energy into electricity.
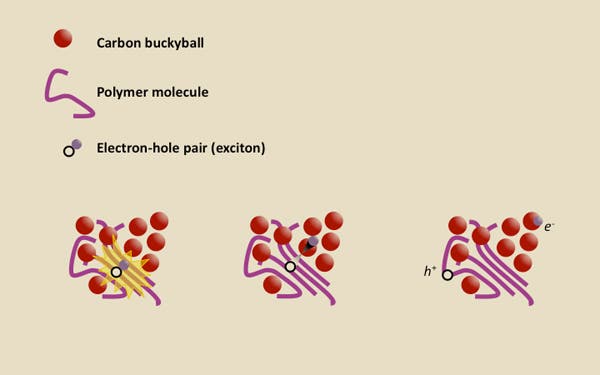
A solar cell, be it made from ordered crystals like silicon or disordered crystals like polymers, converts solar energy carried by photons into electricity. All solar cells contain at least one semiconductor junction called a p-n junction. One semiconductor become negatively charged, while the other become positively charged as a result of incident photon interaction with the semiconducting material. When a photon hits the solar cell, it gets absorbed by the semiconductor material and if its energy is grater than the semiconductor’s band gap, it causes an electron to move leaving what’s referred to as a “hole” instead. The electron then displaces another electron, leaving a new hole, while the previous hole gets filled by another electron and so on – this flow of electrons creates an electrical current. Voila!
Are we doing it all wrong?
This works a bit different in organic solar cells though.
“To generate a current, you have to separate the electron and the hole,” said senior author Alberto Salleo, an associate professor of materials science and engineering at Stanford. “That requires two different semiconducting materials. If the electron is attracted to material B more than material A, it drops into material B. In theory, the electron should remain bound to the hole even after it drops.
“The fundamental question that’s been around a long time is, how does this bound state split?”
The leading theory on how organic solar cells based on polymer semiconductors work is that the electron carries extra energy when it drops from material A to material B. That added energy gives the excited (“hot”) electron enough velocity to escape from the hole. According to Stanford researchers, however, this theory doesn’t necessarily stand when experiments are made.

For instance, several groups expected to attain significant results by redesigning pliant polymers of plastic into orderly, silicon-like crystals, but no significant improvement was seen. The Stanford researchers took polymer semiconductor solar cells to the SLAC National Accelerator Laboratory where they performed X-ray analysis. The X-rays revealed a molecular structure resembling a fingerprint gone awry. Some polymers looked like amorphous strands of spaghetti, while others formed tiny crystals just a few molecules long.
“In our study, we found that the hot exciton effect does not exist,” Salleo said. “We measured optical emissions from the semiconducting materials and found that extra energy is not required to split an exciton.”
What then causes electron-holes to seperate? The Stanford researchers aren’t certain yet, but they believe it has something to do with the the chaotic polymer structure. By analyzing light emissions from electricity flowing through the samples, the Stanford team determined that numerous small crystals were scattered throughout the material and connected by long polymer chains, like beads in a necklace. The small size of the crystals was a crucial factor in improving overall performance, Salleo said.
“Being small enables a charged electron to go through one crystal and rapidly move on to the next one,” he said. “The long polymer chain then carries the electron quickly through the material. That explains why they have a much higher charge mobility than larger, unconnected crystals.”
In their study, Salleo and team found that disorder at the molecular level actually improves the performance of semiconducting polymers in solar cells. In their experiments, the researchers reached an efficiency of 9% which is very high for these types of solar cells. Next the researchers plan on improving these results by better designing the order-disorder polymer interface.
“In organic solar cells, the interface is always more disordered than the area further away,” Salleo explained. “That creates a natural gradient that sucks the electron from the disordered regions into the ordered regions. “
If they measure considerable success in the future attempts, then a paradigm shift may occur. The work was reported in a paper published in the journal Nature Materials.