In 1974, the late Stephen Hawking argued in a now-famous study that besides mass and spin, black holes can be characterized by a unique temperature. He also claimed that black holes don’t just devour matter, but also emit radiation. Researchers at the Israel Institute of Technology have set out to test this theory by creating a black hole analog in the lab. The results agreed with Hawking’s predictions, giving more credence to the theory.
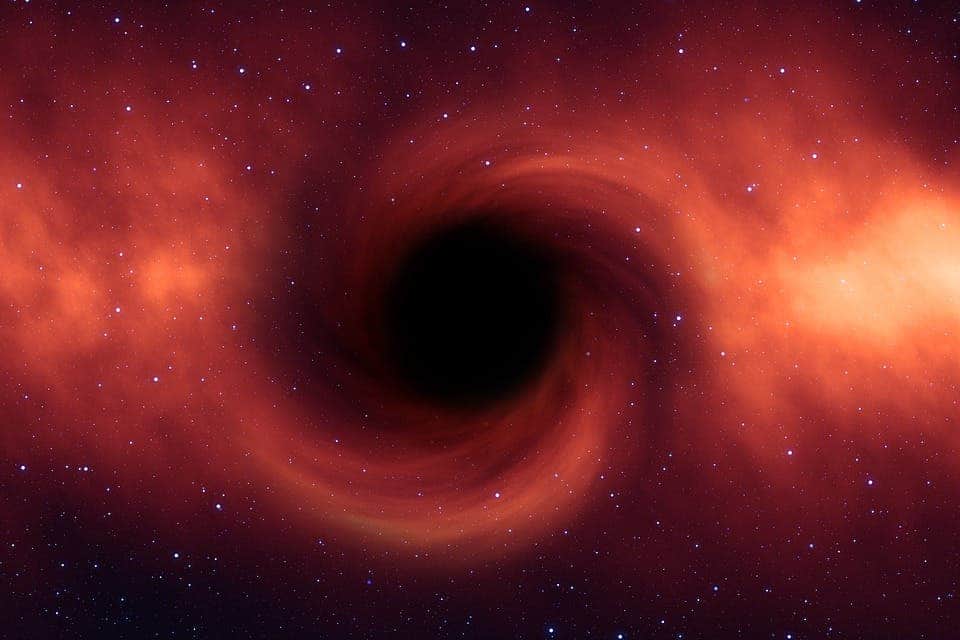
A black hole is defined as a region of spacetime whose extremely strong gravity prevents anything, including light, from escaping. This essentially means that we can’t see a black hole directly — although this year astronomers captured a picture of a black hole’s event horizon (the swirling, bright boundary of the black hole). Scientists are confident that black holes exist, judging from their theoretical calculations and observations of X-rays emitted by swirling disks of gas around the black hole. The motions of nearby stars can also infer the presence of a black hole. In fact, most galaxies — the Milky Way included — are thought to be held together by the gravity of supermassive black holes (with masses millions of times that of the sun), which lie at the galactic center.
But if everything gets sucked into a black hole, never to return, what happens to the information that these objects used to hold? According to the laws of quantum mechanics, matter cannot simply disappear without leaving behind information of its previous state. So, on the one hand, we have physics that says information is never truly lost, nor is it truly copied, while on the other hand, we know that an object that gets too close, and crosses the black hole’s event horizon, it can never escape.
This is known as the black hole information paradox — and Stephen Hawking had been trying to crack it for decades. His investigations eventually led him to develop the Hawking radiation theory, in which the physicist argued that not all matter falls into a black hole. In some cases, when entangled pairs of particles are attracted into a black hole, only one of them would fall in, while the other escaped. Hawking named these escaping particles Hawking radiation, theorizing that its nature should be thermal radiation whose temperature would depend on the size of the black hole.
Testing such a theory is virtually impossible because we currently lack the technology required to measure the radiation from a real black hole. Which is why a team of researchers at the Israel Institute of Technology had to come up with a creative solution. For their new study, the authors made a Bose-Einstein condensate (BEC) — the fifth form of matter where familiar physics fades away, and quantum phenomena start to take over, even at a macroscopic scale. BEC matter almost stops behaving as particles and starts behaving more like waves. In a BEC you can observe “waves of atoms”, moving synchronized with each other just like water drops in an ocean wave.

To make BEC, the Israeli researchers trapped 8,000 rubidium atoms in a focused laser beam, chilling matter to only a billionth of a degree above absolute zero. A second laser fired on one side of the BEC made it denser on that side. According to the researchers, this led to a transition that moves at a constant speed through the condensate from the denser area (outside of the black hole) to the less dense area (analogous to the inside of the black hole). This is where it gets a bit tricky: the researchers say that sound waves traveling through the denser region move faster than this transitional flow, allowing sound to move in either direction. However, in the less dense region, sound waves can only travel away from the sharp transition — in other words, further into the black hole analog.
Light can either move away or into a black hole (and never escape) and in this experiment, light was replaced by sound. In experiments, researchers forced one of a pair of phonons (like photons for sound) to fall into the flow of rubidium atoms, while the other was allowed to escape. When the researchers measured both phonons, they recorded an average temperature of .035 billionths of a Kelvin, which agrees with Hawking’s predictions.
These findings in no way prove Hawking’s theory — that would require technology that doesn’t currently exist. However, the study published in Nature shows that Hawking was definitely on to something.