The secrets of the Quark-Gluon Plasma that filled the early universe are being unlocked by the ALICE collaboration at CERN with the first measurement of the flow of bottomonium particles.
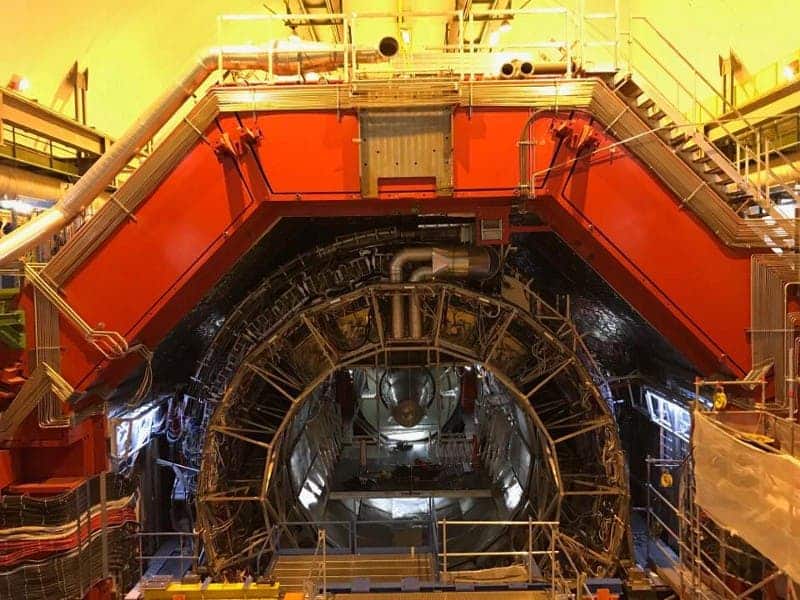
In a paper presented at the European Physical Society’s conference on high-energy physics, the ‘A Large Ion Collider Experiment’ (ALICE) collaboration has documented the first-ever measurement of the flow of a heavy meson particle — bottomonium.
The measurement of particles like bottomonium — a type of ‘upsilon’ particle — helps the researchers understand the Quark-Gluon Plasma (QGP) that filled the hot, dense early universe.
By observing pairs of ‘heavy electrons’ — known as muons — produced by the decay of bottomonium, the team discovered bottomonium particles have small values of elliptic flow — a measure of how uniform energy and momentum is distributed across the particles when viewed from the beamline.
This is quite unexpected as all other hadrons investigated thus far have exhibited significant elliptic flow.
David Evans, a professor of high energy physics at the University of Birmingham, leads the UK participation in ALICE.
He says: “ Elliptical flow measurements in ALICE show that the Quark-Gluon Plasma flows like an almost perfect liquid, with the light quarks (up, down, strange, and charm) flowing with the system.”
“The fact that no significant elliptical flow is seen for the bottomonium suggests b-quarks are only produced in the initial collision of the lead ions, before the QGP is formed.”
The ALICE team’s results seem to support existing theories that bottonium and other upsilon particles split into their constituents during the early stages of their interactions with plasma.
This gives the researchers a better insight at the conditions in the early moments of the universe when it was filled with a plasma composed of free quarks and gluons.
Evans continues: “This makes b-quarks (and particles made up of b-quarks) an ideal probe for studying the QGP as they experience the entire evolution of the system.”
Bottomonium particles — probing the Quark-Gluon Plasma at the dawn of the universe
Taking a trip through the ‘particle zoo’ to discover what these bottomonium particles actually are, helps us understand their role in the ALICE experiment.
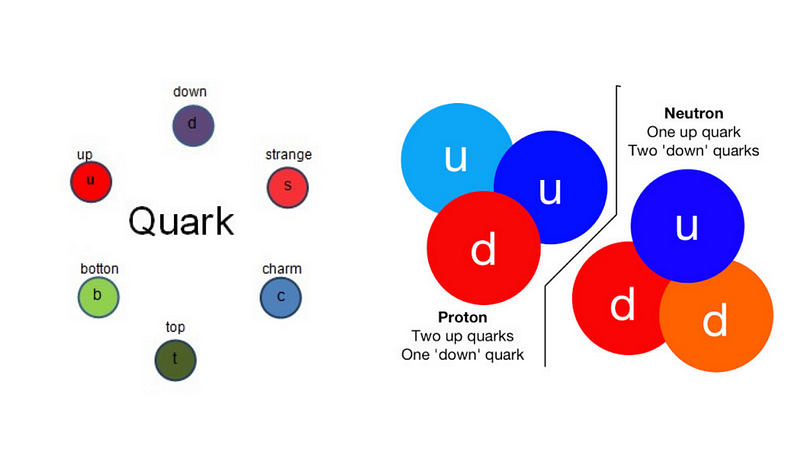
Bottonium is a heavy meson — particles which consist of a quark and its own antiparticle. In the case of bottomonium, a bottom (or beauty quark) — b quark — and its antiparticle counterpart.
These subatomic particles are extremely unstable, existing for short periods of time and only at high energies before decaying into other particles. When bottomonium decays it leaves behind a pair of ‘heavy electrons’ called muons.
Bottomonium particles — which are formed in the LHC by the violent collision of heavy lead-lead ions — provide an excellent probe of the Quark-Gluon Plasma which filled the universe just a few millionths of a second after the big bang.
Being produced so early in the collision event means bottomonium particles ‘experience’ the entire evolution of the plasma — from the moment it is produced to the moment it cools down and enters a state in which hadrons can form.
This extremely early stage in the universe’s evolution would have been the only time in history that quarks and gluons existed freely in plasma and not bound together in a state called ‘confinement’ in protons, neutrons and other hadrons.
These particles were only able to exist in this free state because of the incredible heat in the universe at this point. In our era, quarks and gluons are never observed as free particles.
Thus, it takes a tremendous amount of energy, to recreate these huge temperatures. At the moment, the Large Hadron Collider (LHC) is the only piece of apparatus on Earth capable of doing this, with collisions in the LHC able to generate temperatures a 100 thousand times hotter than the Sun.
At these temperatures, protons and neutrons ‘melt’, freeing quarks and gluons from confinement, thus creating a Quark-Gluon Plasma and allowing them to form short-lived, unstable particles like bottonium.
ALICE: Collision queen
The ALICE collaboration — consisting of over a 1000 scientists operating a 10-thousand-tonne, 16m tall detector buried 56m under the Alps — achieves this high-energy feat by slamming together beams of lead ions rather than the proton-proton collisions used in other LHC experiments.
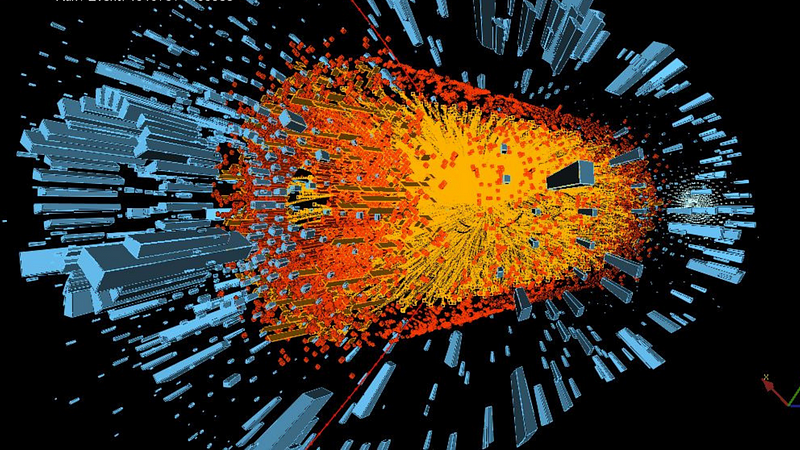
The benefit of colliding lead ions is that, as ALICE is looking to create Quark-Gluon Plasma, the more quarks available to it, the better the chance of observing something significant.
A single proton and neutron each contain three quarks but as lead ions contain at least 56 protons and at least 204 neutrons this leaves the team with far more quarks to play with.
ALICE then measures this plasma as it expands and cools, but it is still unable to measure the particles created directly— instead deducing the presence and properties of QGP from the signatures on pairs of muons it produced by decay.
One of these signatures is the elliptic flow — the collective movement of the produced particles determined by several factors like particle type, mass, the angle at which the particles meet and the momentum they possess as they collide — which is what the team measured. The flow is created by the expansion of hot plasma after the collision of the lead ions.
Upgrading ALICE: More collisions. More Quarks. More Results
Perhaps the most promising thing about ALICE’s mission to probe the early universe and its conditions is the fact that the forthcoming high-luminosity upgrade only promises to yield more data for researchers to investigate.
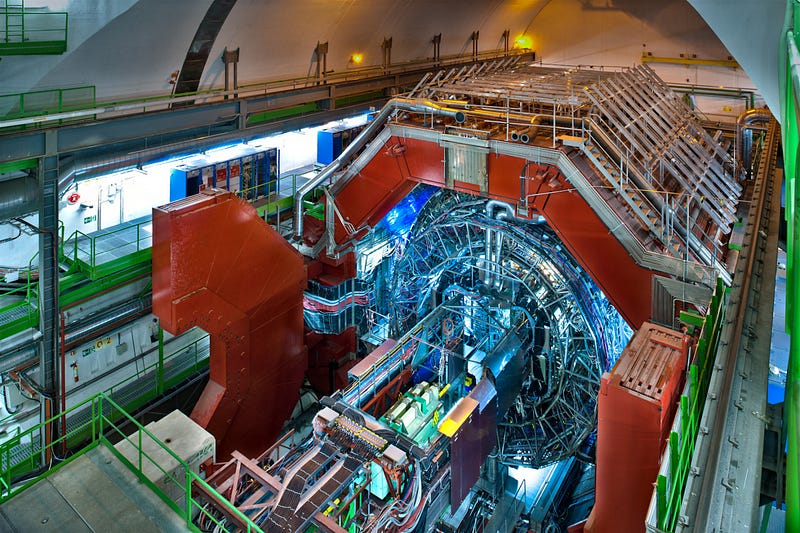
The upgrade — which CERN hopes will be operational by 2021— aims to increase the luminosity of the LHC by a factor of 10. Luminosity, as described in reference to particle accelerators, is proportional to the number of collisions that occur. Thus increasing the luminosity also means increasing the number of collisions.
As an example of the usefulness of this upgrade, whereas the LHC produced 3 million Higgs boson particles in 2017, the High-Luminosity LHC is expected to produce 15 million per year.
In addition to the High-Luminosity upgrade — on which work began in 2018 — ALICE will also several other upgrades and improvements. As a result of these improvements, the ALICE team expect an overall gain of 100 times the current results.
Evans says: “With this huge increase in statistics and a new inner detector in ALICE, we will be able to measure particles made of b-quarks with much higher precision.”
As such, the experiment stands a very good chance of significantly improving our knowledge of the quark-gluon plasma and the conditions in the early universe, with these new bottomonium results pointing the way.
Evans concludes: “[The upgrades] allow us to probe the properties of the QGP in much more detail and hence learn more about the evolution of the early universe.”