A crystal known to science for more than a century was only recently recognized for its use in harvesting solar power. Since the first successful usage of perovskite in solar cells in 2009, the advances in the field have grown exponentially over time, making it a potential candidate for revamping the solar industry in favor of silicon. Despite solar cells made with perovskite recently crossed the 20 percent efficiency mark, researchers say there’s still room to improve if only they knew how charge flows at the nanometer scale. They just had to ask, and the Department of Energy’s Oak Ridge National Laboratory delivered.
Why you should care about perovskite
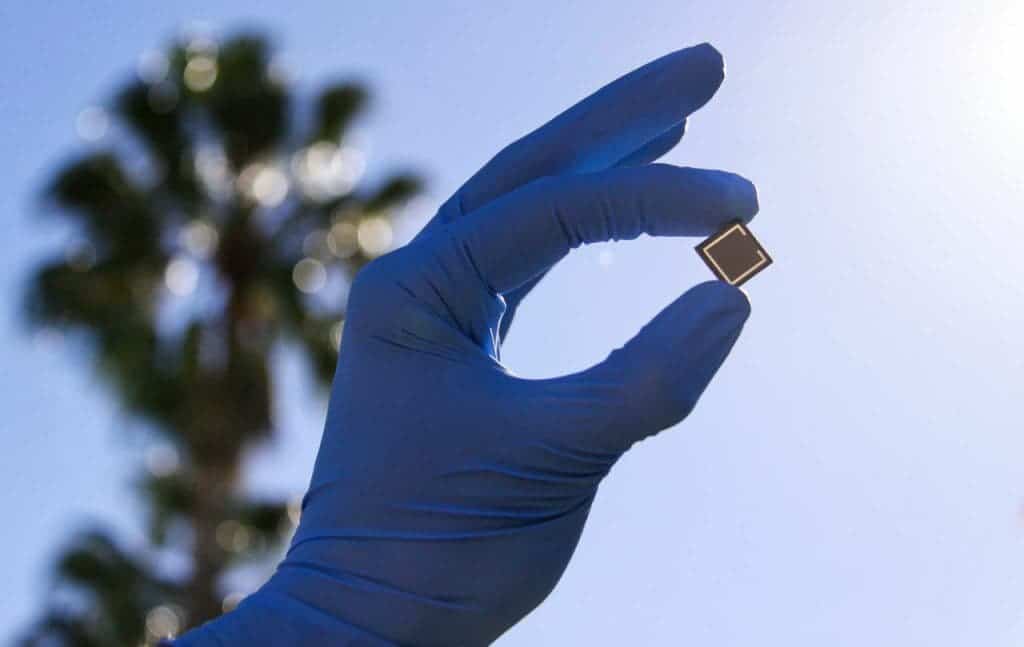
The perovskite mineral was originally found in the Ural Mountains in 1839, but it was only a few years ago that its ability to transport solar energy and convert it into electricity was discovered. Just a couple of years later, rated efficiency in the lab has soared from 3.8% to 19.3%, a pace of improvement unmatched by any other solar technology. Currently, the leading commercial solar tech employs crystalline silicon solar cells, which convert roughly 25% of incoming photon energy into electricity, but have decades worth of research backing them.
What makes this mineral so exciting is its uncanny ability to diffuse photons a long distance through the cell when prepared in a liquid solution. Typically, solar cells convert energy to electricity by exploiting the hole-pair phenomenon. The photon hits the semiconducting material, then if its energy falls into the semiconductor bandcamp, an electron is offset, leaving a gap in the atom or hole. The electron travels from atom to atom within the material, occupying holes and offsetting at the same time until it eventually reaches an electrode and has its charge transferred to a circuit. Last step: profit and generate electricity.
The key is to have electron moving for as long as possible, and thanks to its diffusing capabilities, perovskite can theoretically generate more electricity. Perovskite is also dirt cheap which is highly important if we’re ever to cover a significant portion of the planet’s surface with solar cells for a 100% sustainable energy future.
Zooming in on charges

An experimental setup combined microscopy and ultra-short laser pulses that fire every 50 millionths of a billionth of a second. These pulses mimicked the sun’s rays by providing photons that got absorbed by a perovskite solar cell. A second laser measured minute changes in light absorption in the material. Eventually, the researchers drew a pixel-by-pixel map of the material.
“The ability to identify what will be created after the solar cell absorbs a photon, either a pair of free charges or their bound form called an exciton, is crucial from both fundamental and applied perspectives,” said co-author Yingzhong Ma, who led the research team. “We found that both free charges and excitons are present, and the strength of our approach lies in not only identifying where they are but also determining what their relative contributions are when they are both present at a given spatial location.”
“With conventional approaches of studying photovoltaic materials, we are unable to accurately map out electronic processes and how electrons are getting lost,” said said Benjamin Doughty, one of the authors and a member of ORNL’s Chemical Sciences Division. “Those processes can translate into losses in efficiency.”
Now, it only remains to identify what causes this spatial difference, Ma says. If and when this happens, they might also learn what causes the degradation issues due to moisture many have reported.
Reference: “Separation of Distinct Photoexcitation Species in Femtosecond Transient Absorption Microscopy,”http://pubs.acs.org/doi/abs/10.1021/acsphotonics.5b00638.