All models of particle physics are based on the mundane assumption that matter and anti-matter behave indistinguishably, but we can’t be sure. Luckily, an experiment at Brookhaven National Lab seems to confirm this basic caveat of particle physics after it found the attractive forces between antiprotons are the same as those seen in regular matter.
The quest for antimatter

For every type of matter particle we’ve found, there also exists a corresponding antimatter particle, or antiparticle. These should look and behave just like their corresponding matter particles, except with opposite charge. A proton is naturally positively charged, as thought in any basic chemistry or physics class. The antiproton, however, is negatively charged. When matter and anti-matter these annihilate each other, releasing energy in the process.
During the Big Bang, matter and antimatter were created in equal amounts, but that’s clearly not what we’re seeing today. In fact, it’s difficult if not impossible to detect antimatter outside of a laboratory setting. For that matter, we wouldn’t have existed in the first place if antimatter and matter were still in equal proportion, given their tendency to annihilate each other. There has to be an explanation, but at this point opinions are mixed.
Some physicists think that after the Big Bang, fractions of a second in, all matter and antimatter canceled each other out but in the process created radiation. Out of this radiation new matter-antimatter pairs were formed, which again annihilated each other creating new radiation and so on. When the universe expanded and cooled to below the temperature where particle-antiparticle pair production could happen, all the antimatter and matter that were in equal proportions annihilated with each other, leaving only radiation. Here’s the kick though. It may be that matter and antimatter weren’t created equal. There is a tiny, *tiny* chance that you only get matter when you try to create matter and antimatter or one particle of matter for every billion annihilation event. As the universe evolved after the Big Bang, these very small symmetry violations may have resulted in the abundance of matter and the dearth of antimatter we see today.
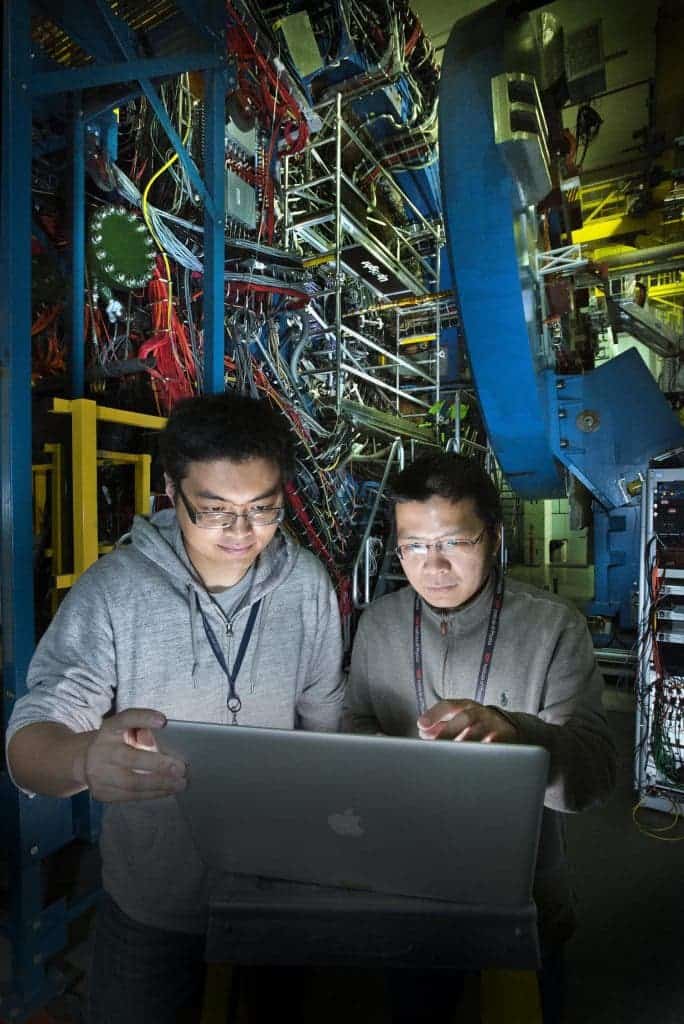
We can’t don’t this for certain yet. “Although this puzzle has been known for decades and little clues have emerged, it remains one of the big challenges of science. Anything we learn about the nature of antimatter can potentially contribute to solving this puzzle,” says Aihong Tang, a Brookhaven physicist.
Tang was involved with Relativistic Heavy Ion Collider where he and colleagues smashed accelerated gold ions together at high energy and relativistic speeds. When the gold ions smashed instead of forming new gold particles, the collision created mostly forms of hydrogen and helium, but also exotic particles like heavy quarks or their antimatter counterparts.
“We see lots of protons, the basic building blocks of conventional atoms, coming out, and we see almost equal numbers of antiprotons,” said Zhengqiao Zhang, a graduate student in Professor Yu-Gang Ma’s group from the Shanghai Institute of Applied Physics of the Chinese Academy of Sciences, who works under the guidance of Tang when at Brookhaven. “The antiprotons look just like familiar protons, but because they are antimatter, they have a negative charge instead of positive, so they curve the opposite way in the magnetic field of the detector.”
“By looking at those that strike near one another on the detector, we can measure correlations in certain properties that give us insight into the force between pairs of antiprotons, including its strength and the range over which it acts,” he added.
Ultimately, the researchers were able to investigate the effective and scattering range of two antiprotons. The effective range between two particles is a measure of how close they have to be to influence each other with their electric charge, while the scattering range is a measure of how much these particles deviate as they travel from source to destination. During this experiment the the scattering length was around 7.41 femtometers and the effective range was 2.14 femtometer, which is roughly the same as the those of a proton pair. Whether matter or antimatter, it seems these types of interactions are virtually indistinguishable.
“This discovery isn’t a surprise,” said Kefeng Xin, a graduate student at Rice. “We’ve been studying the interaction between nucleons (particles that make up an atom’s nucleus) for decades, and we’ve always thought the forces between antimatter particles are the same as for matter. But this is the first time we’ve been able to quantify it.”
“There are many ways to test for matter/antimatter asymmetry, and there are more precise tests, but in addition to precision, it’s important to test it in qualitatively different ways. This experiment was a qualitatively new test,” said Richard Lednický, a STAR scientist from the Joint Institute for Nuclear Research, Dubna, and the Institute of Physics, Czech Academy of Sciences, Prague.
“The successful implementation of the technique used in this analysis opens an exciting possibility for exploring details of the strong interaction between other abundantly produced particle species,” he said, noting that RHIC and the Large Hadron Collider (LHC) are ideally suited for these measurements, which are difficult to assess by other means.
We’ve yet to solve the puzzle. Namely, we still don’t know why there’s so little antimatter left in the universe, but at least we now know that it’s not due to some difference in how the two types of matter interact.