In vacuum, light always travels at a constant speed of 299,792,458 metres per second. Nothing can travel faster than this constant c, as denoted by physicists. These two postulates are basic building blocks of modern physics and were first announced more than a hundred years ago by Albert Einstein.
Yes, yes nothing can travel faster than light, but…
When light travels through a medium other than vacuum, it will be slowed down. For instance, when light propagates through water or air, it will do so at a slower speed. That’s due to the fact that light scatters off the molecules that make-up different materials. The photons themselves do not slow down. But their passage through a medium involves absorption by electrons and re-emission. For some materials such as water, light will slow down more than electrons will. Thus, an electron in water can travel faster than light in water. But nothing ever travels faster than c.

In some instances, sluggish light can produce some very interesting physical phenomena. You probably heard about the sonic boom. A ‘normal’ subsonic aircraft will deflect air smoothly around its wings. A supersonic aircraft — the kind that travels faster than sound (more than 340 m/s) — will actually move way faster than the air it dislocates. The result is a sudden pressure change or shock wave which propagates away from the aircraft in a cone at the speed of sound.
Dr. Manhattan
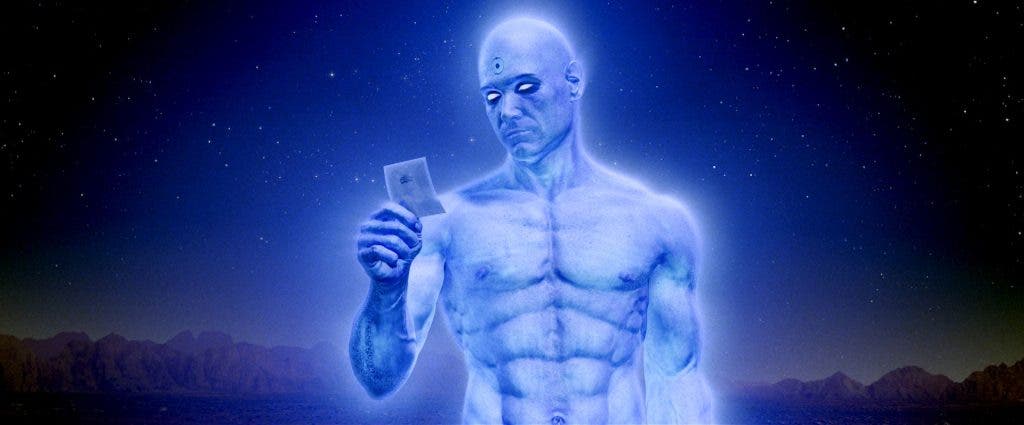
The phase velocity of light in a medium with refractive index n is vlight = c/n. Water has a refractive index of about 1.3, so the speed of light in water is considerably less than the speed of light in vacuum. Not only an electron can move faster than light through a different medium — other particles as well. If a charged particle travels faster than light in a medium, than a faint radiation is produced. In water, for example, the charged particle excites the water molecules, which then return to their normal state by emitting photons of blue light. The light propagates in a cone forward of the region where the interaction took place, analogous to the sonic boom.
This effect, known as Cherenkov radiation, was observed as a faint blue glow by Pavel Cherenkov in 1934 when he was asked to look at the effects of radioactivity in liquids. People working with nuclear reactors often get to see this telltale blue glow. In popular culture, the powerful Doctor Manhattan from lan Moore’s classic “Watchmen” graphic novel is always surrounded by a blue glow.
This discussion begs the question: how much can we slow light?
Dead stop
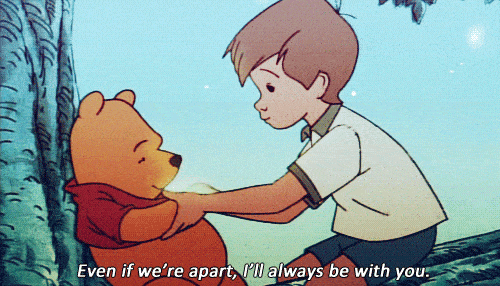
While we can never actually speed up or reduce the speed of light, which is always a constant, scientists have been successful in manipulating the time it takes for light to travel through various mediums. At room temperature, atoms are incredibly fast and behave akin to billiard balls, bouncing off each other when they interact. As you lower the temperature (remember temperature reflects atomic agitation), atoms and molecules move slower. Eventually, once you get to about 0.000001 degrees above absolute zero, atoms become so densely packed they behave like one super atom, acting in unison. This is the domain of quantum mechanics so prepared for a lot of weirdness.
This is actually a distinct state of matter known as the Bose-Einstein condensate, which doesn’t resemble everyday observable states like liquid, gas, solid or plasma. BEC, for short, was first predicted in the 1920s by Albert Einstein and the Indian physicist Satyendra Bose and it wasn’t until very late in 1995 that scientists were able to produce the necessary conditions for this extreme state of matter to occur.
In 1999, Lene Vestergaard Hau, a professor at Harvard University, aimed a laser beam through such a cloud of nearly motionless sodium atoms only 1/125 inch long. First, an initial beam known as the coupling beam is shone on the cloud rendering it transparent. It does with an extremely fast rate of the change of the refractive index.
A second laser beam, the probe pulse, fires through this now-transparent cloud of gas which has a refraction index a hundred trillion times higher than that of glass in optical fiber. It was under these conditions that light crawled at a staggering 38 miles per hour. Horses are faster.
Floating light
Not resting on her laurels, Professor Hau pushed the envelope to the ultimate point: stopping light in its tracks. To stop light altogether, the scientists utilized a similar but far more powerful effect. The researchers cooled a gas of magnetically trapped sodium atoms to within a few millionths of a degree of absolute zero (-273 deg C). The experimental setup looked very similar to the first attempt, only this time if the team turned off the coupling laser while the probe laser was still shining on the cloud, then the probe pulse would stop dead. If the coupling beam is then turned back on, the probe pulse emerges intact, just as if it had been waiting to resume its journey. Astonishing! These findings were replicated in the same year (2001) by Dr Ronald Walsworth, of the Harvard-Smithsonian Center for Astrophysics, Cambridge.

Since then, various milestones were set. In 2013, a team from Germany’s University of Darmstadt put light to a full stop inside a crystalline structure and kept it so for a full minute. They also used the trap to store and then retrieve an image consisting of three stripes. “We showed you can imprint complex information on your light beam,” said lead researcher George Heinze. In 2015, researchers at University of Glasgow found a way to slow the speed of light that does not involve running it through a medium. They essentially altered its speed indirectly by running the light through a special mask — a filter that shaped the beam into either a Gaussian or Bessel beam.
After it passes through a medium, say glass, water or any kind of material that you can make a filter out of, light is supposed to speed back up to its normal constant. The experiment showed that light can be caused to travel slower than c, by changing its shape. This was 0.001 percent slower than it should have. Not as impressive as putting the brakes on light, but still fascinating. Maybe light is more malleable than physicists previously thought.
There are, of course, practical applications to these ‘gimmicks’. These include quantum computing and quantum communication applications. Still, doing this sort of groundbreaking science just for the sake of it sounds just as awesome to me.