Geophysicists trying to understand hotspot volcanoes have used a process known as seismic tomography and detected previously unknown finger-like structures of heat, some thousands of km long.

The vast majority of volcanoes arises at contact zones between tectonic plates. However, another, entirely different type of volcano exists: hotspots are volcanic regions which can appear even in the middle of tectonic plates. It is believed that they are ‘fed’ by underlying mantle that is anomalously hot compared with the mantle elsewhere and rises due to this temperature difference (just like hot air rises above cold air).
But some hotspot volcanoes cannot be explained by this process – something which suggests significantly more complex interactios between these hot plumes and the upper mantle. It is even believed that this type of volcanoes, if understood, could provide models of interaction for the entire mantle. This is why University of Maryland seismologist Vedran Lekic and colleagues at the University of California Berkeley developed a new computer modelling approach to this phenomena.

Earthquakes and seismic waves
Everytime there is an earthquake (or significant volcanic eruption, or even a big explosion), seismic waves are emitted. They are a type of acoustic wave and are constantly monitored by a global network of seismographs; this is how they monitor any nuclear events as well. As seismic waves pass through different layers from the source (earthquake) to the seismograph, they change their speed and shape depending on the parameters of the environment they are in. By comparing the waveforms from hundreds of earthquakes recorded at locations around the world, seismologists can make deduction about the structures through which the seismic waves have traveled.
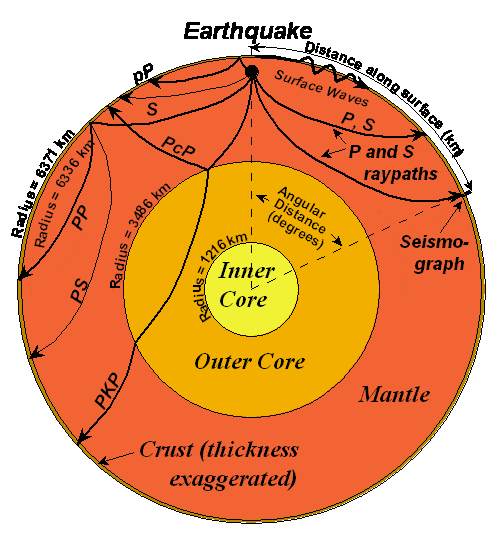
This process is commonly known as seismic tomography, and as the name says, it works basically on the same principle as CT scans (computed tomography). But the problem is that we know so much less about the inside of the Earth than we do about the human body, so interpreting the data from seismic tomography is a titanic job.
“The Earth’s crust varies a lot, and being able to represent that variation is difficult, much less the structure deeper below” said Lekic, an assistant professor of geology at the College Park campus.
This process involves dreadfully complicated mathematics and a process of trial and error – until recently, it would have taken almost 20 years to conduct the necessary calculations for a study like this. But UC Berkeley Prof. Barbara Romanowicz developed a method to more accurately model waveform, while also drastically reducing computing time.
Hot Results
Using this method for interpreting the data, geophysicists reached the conclusion that long fingers of heat lie in the upper mantle (seismic waves move slower in hotter media).
“We estimate that the slowdown we’re seeing could represent a temperature increase of up to 200 degrees Celsius,” or about 390 degrees Fahrenheit, said French, the study’s study lead author. At these depths, absolute temperatures in the mantle are about 1,300 degrees Celsius, or 2,400 degrees Fahrenheit, the researchers said.
Their findings fit perfectly with previous geophysical predictions. But the new images reveal for the first time the extent, depth and shape of these channels. They also showed something rather unexpected: these ‘fingers’ are interacting with tectonic plate movement, and almost certainly affect the plumes rising up to form hotspots.
“This global pattern of finger-like structures that we’re seeing, which has not been documented before, appears to reflect interactions between the upwelling plumes and the motion of the overlying plates,” Lekic said. “The deflection of the plumes into these finger-like channels represents an intermediate scale of convection in the mantle, between the large-scale circulation that drives plate motions and the smaller scale plumes, which we are now starting to image.”
Journal Reference: Scott French, Vedran Lekic, Barbara Romanowicz. Waveform Tomography Reveals Channeled Flow at the Base of the Oceanic Asthenosphere. Science, 2013 DOI: 10.1126/science.1241514