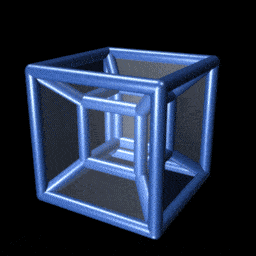
The world around us makes sense in only three spatial dimensions: left and right, up and down, forward and backward. Mathematically, on the other hand, some very clever people have argued that reality may be comprised of at least four spatial dimensions. Modern string theory even goes as far as postulating up to 10 spatial dimensions.
All of these are rather abstract ideas with equally abstract proofs. This is why a recent experiment performed by Swiss and American physicists is so exciting. Their work demonstrates a possible pathway by which higher-dimensional phenomena can be observed in a lower-dimensional system, perhaps even ours.
As you may have guessed already, such experiments are based on our good old ally — quantum mechanics — which never fails to scramble our fragile reality and boggle the mind.
Essentially, each of the two teams, one in the USA, the other in Europe, devised an experimental setup — one with ultra-cold atoms and another with light particles — that allowed them to glimpse the fourth spatial dimension by virtue of the quantum Hall effect; the dynamical version of the effect to be more precise which was previously predicted to occur in 4-D systems.
To understand what these researchers are on about, it helps to picture a more familiar example. When struck by light, a 3-D object will cast a 2-D shadow. Though the shadow doesn’t have depth, you can still learn much about the 3-D object that casts it. For instance, a circle corresponds to a sphere and a square corresponds to a cube. Conversely, a cube can be considered the shadow or 3-D projection of a 4-D object called a hypercube, which we can’t really fathom, just like a 2-D ‘flat-lander’ has no hope of visualizing 3-D. Carl Sagan explained this crucial difference during a historic program.
Bearing all of this in mind, we can consider quantum Hall physics as the “4-D shadow” manifesting itself in 3-D.
The Hall effect occurs when charged particles move in a two-dimensional plane in the presence of a magnetic field. The magnetic field deflects the particles in the direction orthogonal to their motion. This can be interpreted by reading a transverse Hall voltage, which can only take certain quantized values. These values are identical irrespective of the specific properties of the experimental sample. What’s more, scientists have proven that this quantum effect cannot take place in three-dimensional systems.
For decades, physicists were convinced that the Hall effect can only manifest itself in 2-D systems, but more recently there have been voices that claimed a similar effect could also take place in four-dimensional systems. In 4-D, there would be even more remarkable properties including a novel, non-linear Hall current, or so the prediction goes.
Finally, two separate groups of researchers led by Professor Oded Zilberberg at ETH Zürich and Professor Mikael Rechtsman at Penn State have now demonstrated a way to observe physical phenomena proposed to exist in higher-dimensional systems.
One group employed so-called topological charge pumps that simulate the transport of electric charges between rubidium atoms that are trapped by lasers. The cloud of atoms that are cooled down close to absolute zero by the lasers is then placed in a 2D optical lattice created by another set of laser beams that fire at a certain wavelength along two orthogonal directions. The resulting ‘charge pump’ resembles an egg-carton-like “crystal of light” in which the rubidium atoms can move. Finally, yet another laser beam which fires with a different wavelength in each direction helps create a superlattice. By carefully monitoring and analyzing at which positions in the superlattice the atoms are located, scientists demonstrated that the transverse motion of modulation that the atoms predominantly use is the equivalent of the non-linear Hall response. This is the essential feature of the 4D Hall effect.

Another experiment involved passing light through a series of special glassware eerily similar to fiber wire that can control the shape of the light wave. These photonic structures can also reveal the intricate boundary phenomena that accompany this same transverse motion as a result of the 4D quantum Hall effect.
“When it was theorized that the quantum Hall effect could be observed in four-dimensional space,” said Mikael Rechtsman, assistant professor of physics and an author of the paper, “it was considered to be of purely theoretical interest because the real world consists of only three spatial dimensions; it was more or less a curiosity. But, we have now shown that four-dimensional quantum Hall physics can be emulated using photons — particles of light — flowing through an intricately structured piece of glass — a waveguide array.”
Both experiments demonstrate what an effect would look like were it to happen in four dimensions. However, this is not any proof that a genuine four-dimensional system behaves this way.
“Right now, those experiments are still far from any useful application,” Zilberberg admitted.
Though there is no practical application in the short term, this kind of research might help unravel aspects of string theory, according to which many higher dimensions are simply compressed in such a way that only our normal three-dimensional world exists.
Findings appeared in two separate papers, both published in the journal Nature (one and two).