All our modern electronics are based on a class of wonder materials called semiconductors. What makes these so valuable is their ability to free electrons when subjected to an electrical current or when hit by light, becoming mobile and eventually routed and switch through a transistor. It’s the very basis of our digital age, be it solar cells or computers. Now, researchers at UC Berkeley have taken a real-time snapshot of electrons being stripped from silicon’s valence shell for the very first time.
A brief jump
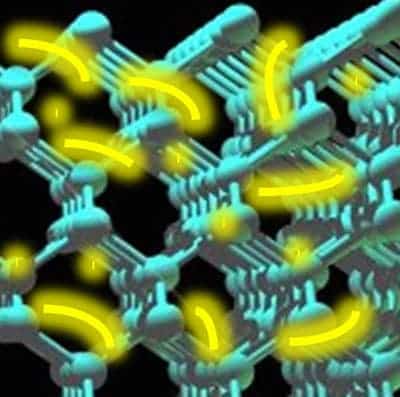
In semiconductors like silicon, electrons attached to atoms in the crystal lattice can be mobilized into the conduction band by light or voltage. Berkeley scientists have taken snapshots of this very brief band-gap jump and timed it at 450 attoseconds. Image: Stephen Leone.
This jump happens so fast that extremely fast lasers, femtosecond lasers, are unable to measure it. This time, scientists turned to a type of laser that sends pulses of light even faster – attosecond pulses of soft X-ray light lasting only a few billionths of a billionth of a second. Experiments show that the time it takes from an electron to transit from the atom’s valence shell, across the band-gap, and into the conduction region is 450 attoseconds or 450 quintillionths of a second.
“Though this excitation step is too fast for traditional experiments, our novel technique allowed us to record individual snapshots that can be composed into a ‘movie’ revealing the timing sequence of the process,” explains Stephen Leone, UC Berkeley professor of chemistry and physics.
In the experiment published in Science, Leone and colleagues zapped a silicon crystal with ultrashort pulses of visible light using a laser. Immediately after the laser was fired, a subsequent X-ray beam was directed which lasted only a few tens of attoseconds (10-18 seconds) to take snapshots of the evolution of the excitation process triggered by the laser pulses. The experimental data was then interpreted by a supercomputer simulation at the at the University of Tsukuba and the Molecular Foundry. Not only did the simulation model the excitation of the electrons, but also the subsequent interaction of X-ray pulses with the silicon crystal.
Physics has identified two distinct states that occur when a semiconducting atom is “activated”. First, the electron absorbs energy and jumps to a higher state where it’s free to roam – it gets excited. Then, the lattice made up of the individual atoms arranged in an orderly manner to form the crystal rearranges itself in response to the redistribution of electrons. In this second stage, part of the energy used to excite the electron is transformed into heat carried by vibrational waves called phonons.
The present experiment confirms this once more, while offering a more refined look of what happens inside. The experiments show that initially, only electrons react to the energy from the laser. Then, after the laser has stopped firing or 60 femtoseconds later, they observed the onset of a collective movement of the atoms, that is, phonons. The researchers estimate the lattice spacing rebounded about 6 picometers (10-12meters) as a result of the electron jump, consistent with other estimates.
“These results represent a clean example of attosecond science applied to a complex and fundamentally important system,” Neumark says.