
We don’t pay too much attention to it, but every whiff of air is a blessing — and we have bacteria to thank. Whilst Earth is a paradise home to countless species of plants and animals, early in its history, our planet looked radically different, with an atmosphere rich in hydrogen sulfide, methane, and ten to 200 times as much carbon dioxide as today’s atmosphere.
Eventually, Earth’s atmosphere transitioned to an oxygen-rich environment conducive to the evolution of complex and diverse life thanks to photosynthetic cyanobacteria. However, this was an arduous and painfully slow process that unfolded over a period of two billion years. Scientists have always struggled to explain why the oxygenation of Earth’s atmosphere took so long.
Now, an international team of researchers has come a step closer to solving this puzzle. In a new study published today in the journal Nature Geoscience, scientists at the University of Michigan, Grand Valley State University, and the Max Planck Institute for Marine Microbiology have proposed a novel model that explains the slow buildup of oxygen content in the atmosphere, linking cyanobacteria oxygenation with a slowdown in Earth’s rotation.
Late rising cyanobacteria

Some of the oldest evidence of life on Earth is 3.5-billion-year-old fossilized remains of microbial mat structures, which look like wrinkle marks in rocks, found in Western Australia. These may have very well been cyanobacteria, also known as blue-green algae. Despite their alternative moniker, cyanobacteria are not actually algae, but rather prokaryotic life forms.
These hardy creatures thrive in hot, cold, salty, acidic, and alkaline environments in which most eukaryotes (organisms with cells that have organelles and distinct nuclei) would perish.
Early cyanobacteria evolved from more primordial organisms and slowly gained the ability to use the sun’s energy, along with carbon and sulfide compounds, to generate energy. The evolution of cyanobacteria went a step further when they started utilizing water during photosynthesis, releasing oxygen as a byproduct.
But although scientists are quite certain cyanobacteria are responsible for the oxygenation of Earth’s atmosphere and the resulting explosion of life, many enigmas remain as to how exactly this process panned out.
“One of the most enduring questions in the Earth Sciences is how Earth became the oxygen-rich planet that we could evolve on. It is clear that photosynthesis is, and always has been, the only significant source of oxygen on our planet. Oxygenic photosynthesis was ‘invented’ by cyanobacteria, the ancestors of all O2 producing phototrophs that we know today. Despite an early evolution of cyanobacteria (before 2.4 billion years ago), Earth only slowly transformed from a reduced harsh environment to the O2 rich planet that we know today. There are many open questions around Earth’s oxygenation pattern. For instance, Why did it take so long after the evolution of oxygenic photosynthesis to reach the first major oxygenation event, the Great Oxidation Event? Why did atmospheric oxygen levels subsequently stay at low levels during the “boring billion” years? What caused the second major rise of oxygen after the boring billion?” geomicrobiologist Judith Klatt of the Max Planck Institute for Marine Microbiology told ZME Science.
The answers to such big questions may have come to Klatt in a flash. Before joining Max Planck, Klat was a postdoc researcher at a lab led by Greg Dick from the University of Michigan. Dick was the leader of an expedition aboard the R/V storm, a 50-foot NOAA research vessel on a mission to collect samples from the Middle Island Sinkhole, several miles offshore from the Michigan town of Alpena.
The water in the Middle Island Sinkhole, found 80-feet-below the water’s surface of Lake Huron, is very rich in sulfur and low in oxygen, conditions that prevailed on Earth billions of years ago.
“This ecosystem thus represents a window into Earth’s past. It, therefore, had been studied already for many years as an analog to the ancient ‘matworld,'” Klatt said.
The mat dance
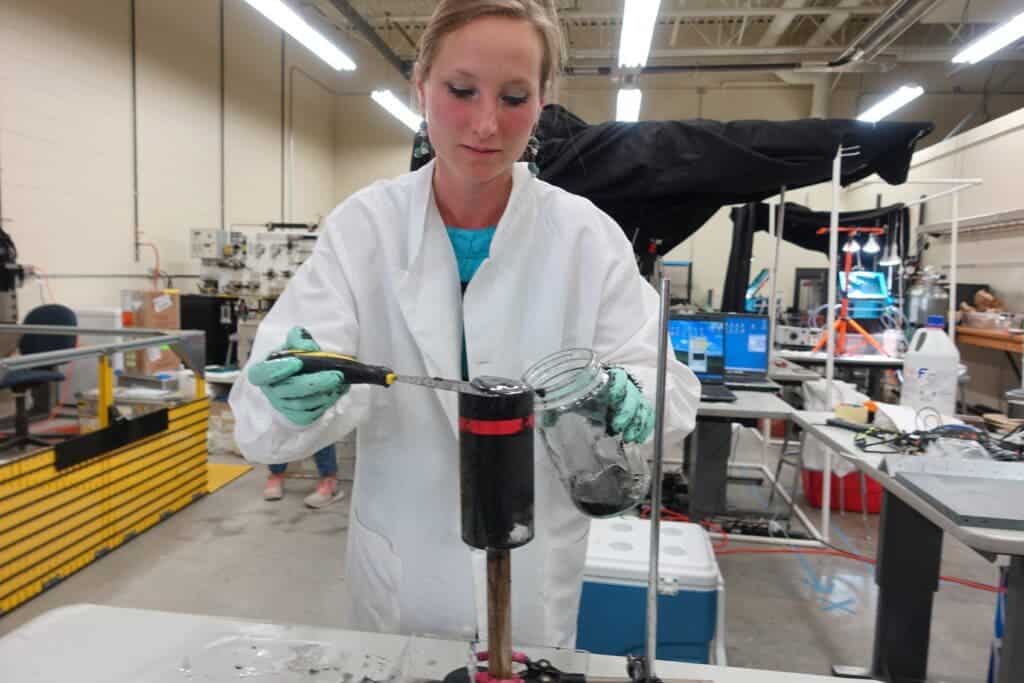
Middle Island Sinkhole (MIS) is inhabited by brightly colored bacteria that thrive where most other creatures would have been doomed. Two types of microbes particularly stand out: purple oxygen-producing cyanobacteria that compete with white sulfur-oxidizing bacteria. The former generates oxygen using sunlight, while the latter eats sulfur for energy.
These microbes have learned to coexist by performing a dance that is repeated and unfolds over the course of each day. From dusk to dawn, the sulfur-consuming bacteria form a mat that sits on top of the cyanobacteria, blocking their access to sunlight. When the sun comes out, the sulfur-eating bacteria descends, making room for the cyanobacteria to rise to the surface and start producing oxygen. However, the cyanobacteria are late risers, taking a few hours before their oxygen production is ramped to the max, Klatt and colleagues learned after performing controlled experiments in Greg Dick’s lab.
“Greg then presented some of these results during a talk for a general audience in 2016. Brian Arbic (a physical oceanographer at the University of Michigan) was present there and then approached us asking if we think that day length would have impacted photosynthesis. While it was clear that MIS mats would have been very sensitive to day length, I was actually hesitant to generalize this thought – despite the stunning similarity between the pattern of oxygenation and Earth rotation rate suggested by some models. It then took more than a year for the next big step. While I was visiting the MPI in Bremen, I suddenly realized that there is in fact a general mechanistic link between day length, i.e. the light dynamics over a day-night cycle, and O2 release from any possible mat. I was super excited, quickly sketched it up, and went over to Arjun Chennu’s desk (who then also worked at the Max Planck Institute for Marine Microbiology and now leads his own group at the Leibniz Centre for Tropical Marine Research (ZMT) in Bremen) to talk it through. He was immediately caught by the idea and we started setting up the numerical model on the very same day. The long journey from tiny to global scales was then a real challenge – but also fun,” Klatt recounted.
Daylight used to be much shorter early in the planet’s history, possibly as short as only six hours. Earth’s rotation rate slowly decreased due to the tug-of-war with the Moon’s gravity and tidal friction. However, this rotational deceleration hasn’t been constant and was even interrupted for about one billion years, which coincides with a long period of static global oxygen levels and stalled biological evolution known as the Boring Billion, a.k.a the Dullest Time in Earth’s History. Earth’s rotational deceleration began again about 600 million years ago, which also marks a major transition in global oxygen concentrations.
To Klatt and colleagues, these coinciding patterns looked more than a simple coincidence. Indeed, the modeling performed by Chennu suggests that day length does, in fact, heavily influence the oxygen release from the cyanobacteria mats.
“After several weeks of modeling, we laid out the oxygen release graphs from two days of different lengths. The difference, while subtle, was there that a longer day releases oxygen differently from a shorter day. We needed much more work to figure out what that means, but I remember that moment when the lines on the graph did not quite align,” Chennu told ZME Science.
These results are somewhat counterintuitive. Although early Earth may have had a 6-hour day, over the span of 24-hours, a surface area would have been exposed to the same amount of cumulative light as today. However, these interruptions caused by early dusk are important owing to molecular diffusion, which is decoupled from oxygen production. In other words, even if the same amount of oxygen is produced by the bacteria during a 24-hour day as during four six-hour days, the net amount of oxygen that is diffused into the atmosphere is much less during the shorter day.
“Even if the mat-cyanobacteria would have produced the very same amount of O2 over billions of years, less O2 would have escaped a mat during a 12 h day compared to an 18-hour day, for instance. These changes might have been relatively small, maybe just a few percent extra oxygen. But considering that Earth was likely a “matworld” these few percent could have had a huge impact. In some extreme cases, including the MIS mats, daylength had, however, a very obvious influence and even turned mats from sinks to sources of oxygen,” Klatt said.
“The heart of our story is simple physics – the physics of diffusional mass transfer, which links the dynamics of light to the export of substances from an ecosystem. When it comes to the question: Could it have mattered sufficiently to shape Earth’s oxygenation pattern?, our story clearly becomes conjectural. We tested our hypothesis as much as possible, but we had to rely on several assumptions, across a wide range of scientific disciplines. Thus, we present a concept rather than a quantitative estimate, which would require more empirical studies,” the researcher added.
Daylight is not the only driver of oxygenation but may have played a crucial role alongside other physical and biological processes, each contributing little but amplifying over time.
“We propose an interaction between planetary mechanics and fine-scale physical and biological processes on extremely small scales over extremely long time scales. The paper is therefore a mosaic of concepts from diverse disciplines. We, therefore, needed to draw from the insights of geology concerning Earth’s oxygenation pattern and from oceanography and astronomy for Earth’s rotational deceleration, for instance. But moving across all kinds of spatial and temporal scales and across fields of research was overall the most fun part,” Klatt concluded.