
Out beyond the orbit of Neptune and the solar system’s seven other major planets lies a ring of icy bodies known as the Kuiper Belt. The disc that is 20 times as wide and an estimated 200 times as dense as the asteroid belt houses a wide array of objects, including its most famous inhabitant — the dwarf planet Pluto. But, it holds more than objects of ice and rock. The Kuiper Belt may hold the secrets of how the planets of the solar system formed, and the raw materials that created the worlds around us and our own planet.
“The Kuiper Belt is a repository of the solar system’s most primordial material and the long-sought nursery from which most short-period comets originate,” explains David C. Jewitt, an astronomer based at the University of California, Los Angeles, who is renowned for his study of the solar system and its smaller bodies. “The scientific impact of the Kuiper Belt has been huge, in many ways reshaping our ideas about the formation and evolution of the Solar System.”
Researchers now stand on the verge of unlocking these secrets with the investigation of the Kuiper Belt contact binary Arrokoth (previously known as ‘Ultima Thule’). On January 2019, the object — named for the Native American word for ‘sky’ — became the most distant object ever visited by a man-made spacecraft.
“Most of what we know about the belt was determined using ground-based telescopes. As a result, Kuiper Belt studies have been limited to objects larger than about 100 km because the smaller ones are too faint to easily detect,” says Jewitt. “Now, 5 years after its flyby of the 2000-km-diameter Kuiper Belt object Pluto, NASA’s New Horizons spacecraft has provided the first close-up look at a small, cold classical Kuiper Belt object.”
The data collected by the New Horizons probe has allowed three separate teams of researchers to conduct the most in-depth investigation of a Kuiper Belt object ever undertaken. In the process, they discovered that our current knowledge of how these objects form is very likely incorrect. From all the evidence the three teams collected, it seems as Kuiper Belts form as a result of a far more delicate, low-velocity process than previously believed. As most astrophysicists believe that these objects — planetesimals — acted as the seeds from which the planets grew, this new model changes our idea of how the solar system formed.
How Kuiper Belt Bodies Get in shape
The majority of the clues as to Arrokoth’s low-velocity formation originate from its unusual binary lobed shape. The larger lobe is joined to the smaller lobe by an extremely narrow ‘neck.’ What is especially interesting about this shape — reminiscent of a bowling pin or a snowman — is that the lobes are perfectly aligned.
John Spencer, Institute Scientist in the Department of Space Studies, Southwest Research Institute in Boulder, Colorado, led a team of researchers that reconstructed Arrokoth’s 3-dimensional shape from a series of high resolution black and white images. Spencer’s paper concludes that Arrokoth’s lobes are much flatter than was previously believed but despite this, both lobes are denser than expected.
William McKinnon, Professor of Earth and Planetary Sciences at the Califonia Institute of Technology, and his team ran simulations of different formation methods to see which conditions led to the shape recreated and Spencer and his colleagues.
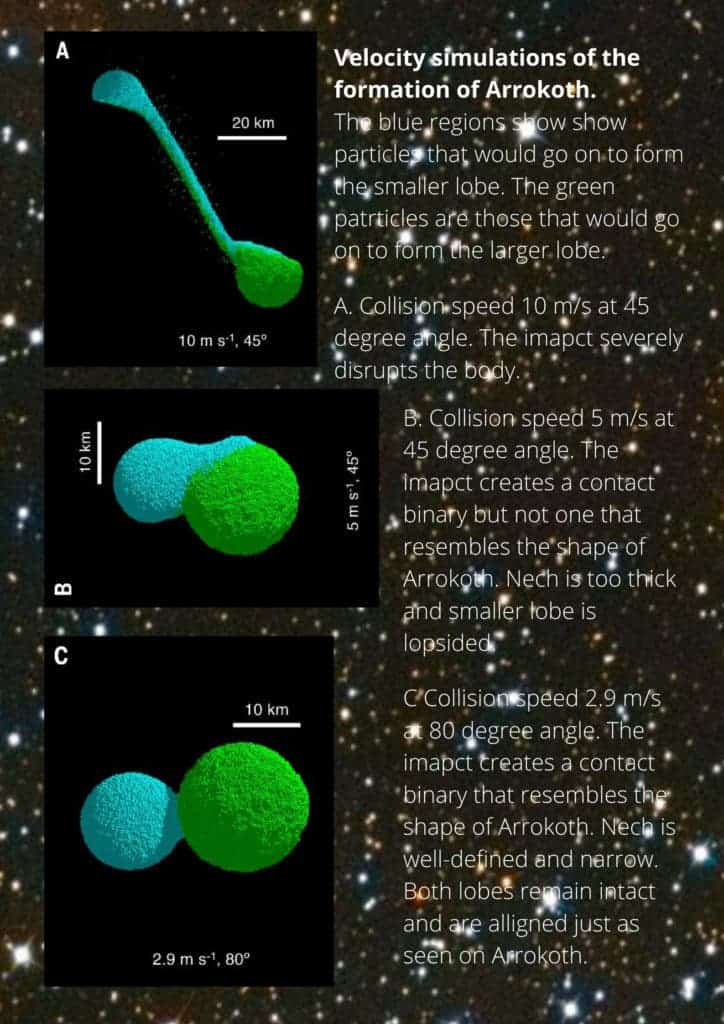
McKinnon and his team discovered that the shape of Arrokoth could only be achieved as a result of a low-velocity formation–around 3 m/s. This presents a problem to current theories of how planetesimals form.
The suggested method of planetesimal formation suggests high-velocity particles smashing together in a process called hierarchical accretion. The simulations that McKinnon produced suggest that such high-velocity collisions would not have created a larger body, but rather, would have blown it apart. The geometrical alignment of the larger and smaller lobes indicates to the team that they were once co-orbiting bodies which gradually lost angular momentum and spiralled together, resulting in a merger.
“Arrokoth’s delicate structure is difficult to reconcile with alternative models in which Arrokoth Kuiper Belt objects are fragments of larger objects shattered by energetic collisions,” Jewitt says. This supports a method of planetesimal formation called ‘cloud collapse.’

“A variety of evidence from Arrokoth points to gravitational collapse as the formation mechanism. The evidence from the shape is probably most compelling,” William Grundy of Lowell Observatory says. “Gravitational collapse is a rapid but gentle process, that only draws material from a small region. Not the much more time consuming and violent process of hierarchical accretion – merging dust grains to make bigger ones, and so on up through pebbles, cobbles, boulders, incrementally larger and larger,
with more and more violent collisions as the things crashing into each other.”
Grundy, whose team analysed the thermal emissions from Arrokoth’s ‘winter’ side, goes on to explain that the speed at which cloud collapse occurs and the fact that all the material that feeds it is local to it means that all the Kuiper planetesimals should be fairly uniform.
Cold Classicals: Untouched and unpolluted
Arrokoth is part of a Kuiper Belt population referred to as ‘cold classicals,’ this particular family of bodies is important to astrophysicists researching the origins of the solar systems. This is because, at their distance from the Sun within the Kuiper Belt, they have remained virtually untouched by both other objects and by the violent radiation of the Sun.
As many of these objects, Arrokoth in particular, date back 4 billion years to the very origin of the solar system, they hold an uncontaminated record of the materials from which the solar system emerged and of the processes at play in its birth.
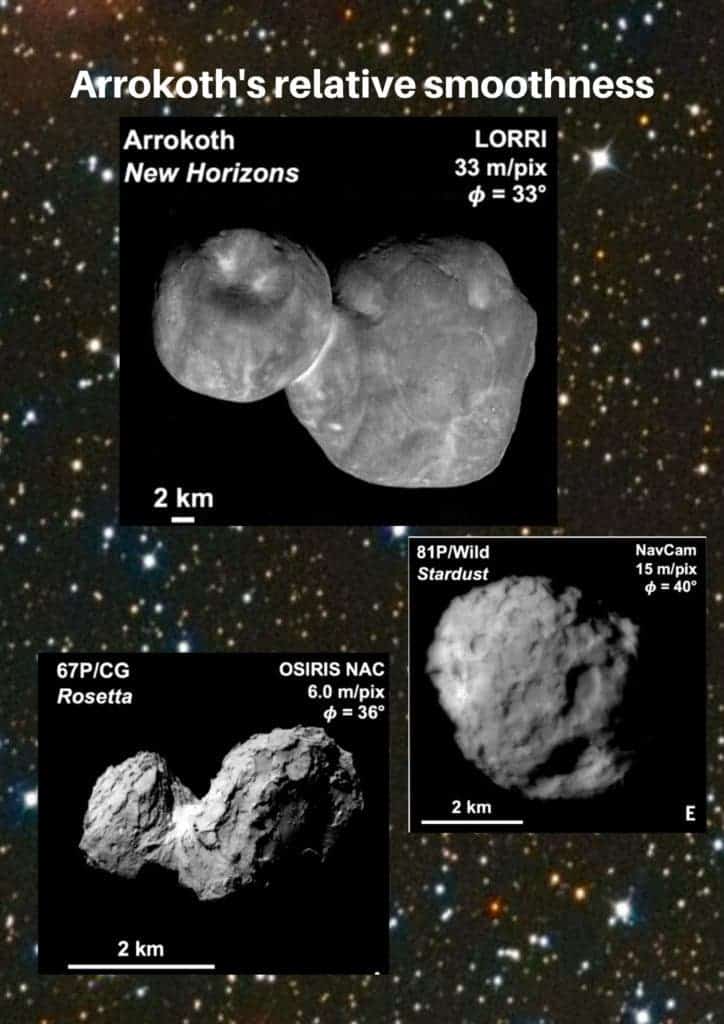
Arrokoth has a relatively smooth surface in comparison with other comets, moons and planets within the solar system. It does show the signs of a few impacts, with one very noticeable 7km wide impact crater located of the smaller lobe. This few craters dotted across Arrokoth’s surface do seem to point to a few small high-velocity impacts. The characteristics of Arrokoth’s cratering allowed the team in infer its age of around 4 billion years. This places its birth right around the time the planets had begun to form in the solar system.
“The smooth, relatively un-cratered surface shows that Arrokoth is relatively pristine, so evidence of its formation hasn’t been destroyed by subsequent collisions,” Spencer explains. “The number of craters nevertheless indicates that the surface is very old, likely dating back to the time of accretion.
“The almost perfect alignment of the two lobes, and the lack of obvious damage where they meet, indicate gentle coalescence of two objects that formed in orbit around each other, something most easily accomplished by local cloud collapse.”
Heatseekers
As mentioned above, Will Grundy and his team were tasked with the analysis of thermal emissions in the radio band emitted by the side of Arrokoth facing away from the Sun.

10.1126/science.aay3705 (2020).
“We looked at the thermal emission at radio wavelengths from
Arrokoth’s winter night side. Arrokoth is very cold, but it does still emit thermal radiation,” Grundy says. “The signal we saw was brighter, corresponding to a warmer temperature than expected for the winter surface temperature. Our hypothesis is that we are seeing emission from below the surface, at depths where the warmth from last summer still lingers.”
Grundy’s team also looked at the colour imaging of Arrokoth with the aim of determining what it is composed of. “We looked at the variation of colour across the surface, finding it to be quite subtle,” he says. “There are variations in overall brightness, but the colour doesn’t change much from place to place, leading us to suspect that the brightness variations are more about regional differences in surface texture than compositional differences.”
The team determined that Arrokoth’s dark red colouration is likely to be a result of the presence of ‘messy’ molecular jumbles of organic materials that occur when radiation drives the construction of increasingly complex molecules–known as tholins.
“One open question is where Arrokoth’s tholins came from,” Grundy says. “Were they already present in the molecular cloud from which the Solar System formed? Did they form in the protoplanetary nebula before Arrokoth accreted? Or did they form after Arrokoth accreted, through radiation from the Sun itself?”
The researcher says that all three are possible, but he considers the uniformity of Arrokoth’s colouration to favour the first two possibilities over the third. The team also searched Arrokoth for more recognisable organic molecules, spotting methanol–albeit frozen solid–but, not finding any trace of water. Something which came as a surprise to Grundy. “It was surprising not to see a clear signature of water ice since that’s such a common material in the outer solar system. Typically, comets have
around 1% methanol, relative to their water ice.”
The team believe that this disparity arises from the fact that Arrokoth accreted in a very distinct chemical environment at the extreme edge of the nebula which collapsed to create the solar system.
“If it was cold enough there for carbon monoxide (CO) and methane (CH4) to freeze as ice onto dust grains, that would enable chemical mechanisms that create methanol and potentially destroy water, too. But those mechanisms could only work where these gases are frozen solid,” Grundy says. “Arrokoth appears to be sampling a region of the nebula where such conditions held.
“We have not seen comets so rich in methanol, which probably means we have not seen comets that formed in this outermost part of the nebula. Most of them probably originally formed closer to the Sun (or else at a different time in nebular history when the chemical conditions were somewhat different).”
Looking to future Kuiper Belt investigations
Investigating Kuiper Belt objects is no walk in the park, with difficulties arising from both the disc’s distance from the Sun and from the fact that Kuiper Belt objects tend to be very small. Grundy explains that as sunlight falls off by the square of its distance, object s as far away as the Kuiper Belt require the most powerful telescopes to do much of anything.
“Sending a spacecraft for a close-up look is great to do, but it took New Horizons 13 years to reach Arrokoth,” Grundy says. “It’ll probably be some time yet before another such object gets visited up-close by a spacecraft.”

“For flybys, the journey times are very long–we flew for 13 years to get there–navigation is difficult because we don’t know the orbits of objects out there very well, we’d only been tracking Arrokoth for 4 years,” Spencer explains. “The round-trip light time is long, which makes controlling the spacecraft more challenging, and light levels are very low, so taking well-exposed, unblurred, images is difficult.”
Spencer adds that from Earth, objects like Arrokoth are mostly very faint, meaning only a small fraction of them have been discovered and learning about their detailed properties is difficult even with large telescopes. These difficulties mean that one of the things left to discover is just how common bi-lobed contact binaries like Arrokoth are in the Kuiper Belt. “Some evidence from lightcurves suggests up to 25% of cold classical could be contact binaries,” he says. ” We know that many of them are binaries composed of two objects orbiting each other, however.”
Fortunately, telescope technology promises to make leaps and bounds over the coming decades, with the launch of the space-based James Webb Space Telescope (JWST) in 2021 and the completion of the Atacama Desert based Extremely Large Telescope (ELT) in 2026.
“Both will help,” says Grundy. “Larger telescopes are needed to collect more light and feed it to more sensitive instruments. JWST and the new generation of extremely large telescopes set to come online over the coming years will enable new investigations of these objects.”
In terms of future spacecraft visits, Grundy believes that researchers and engineers should be thinking small, literally: “If technical advances were to enable highly miniaturized spacecraft to be flown to the Kuiper belt more quickly, that could enable a lot of things. The big obstacles to doing that with today’sCubeSats are power, longevity, and communications, but the rapid advance of technology makes me hopeful that it will be possible to do a whole lot more with tiny little spacecraft within a few decades.
“It’s funny how progress calls for ever bigger telescopes and ever smaller
spacecraft.”
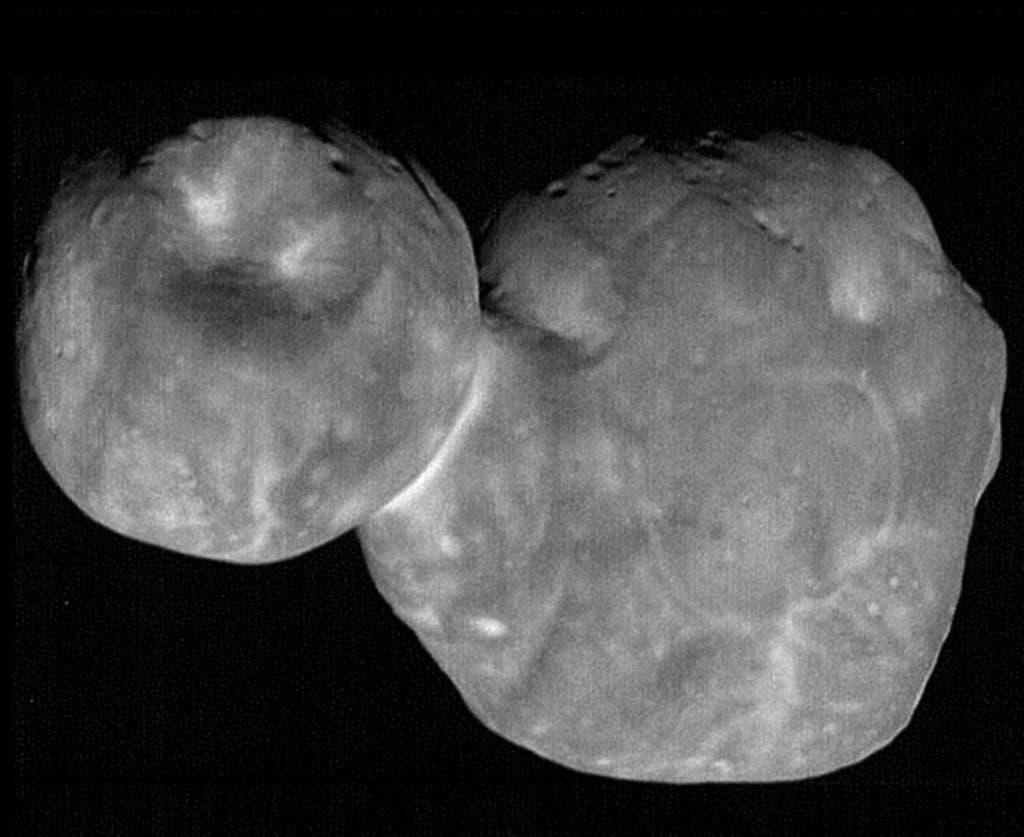
Of course one of the most lasting changes that result from this landmark triad of studies on Arrokoth published in Science is the move away from hierarchical formation models and the adoption of a gravitational or cloud collapse model to explain the creation of planetesimals. This shift will resolve one of the long-standing issues with the hierarchical model, the fact that they work quite well to grow things from dust size to pebble size, but once pebble size is reached, the particles quickly spiral-in toward the Sun.
“I think it will shift the focus to the circumstances that trigger the collapse. It’s a very fast way of making a planetesimal–decades instead of hundreds of millennia–but the circumstances have to be right for instabilities to concentrate solids enough for them to collapse,” Grundy explains. “It will be interesting to map out where and when planetesimals should form, what their size distributions should be, and where the solids that they are formed from should have originated.”
Original research:
W. M. Grundy et al., Science
10.1126/science.aay3705 (2020).
W. B. McKinnon et al.,
Science 10.1126/science.aay6620
J. R. Spencer et al., Science
10.1126/science.aay3999 (2020).
D. C. Jewitt et al., Science
10.1126/science.aba6889 (2020).