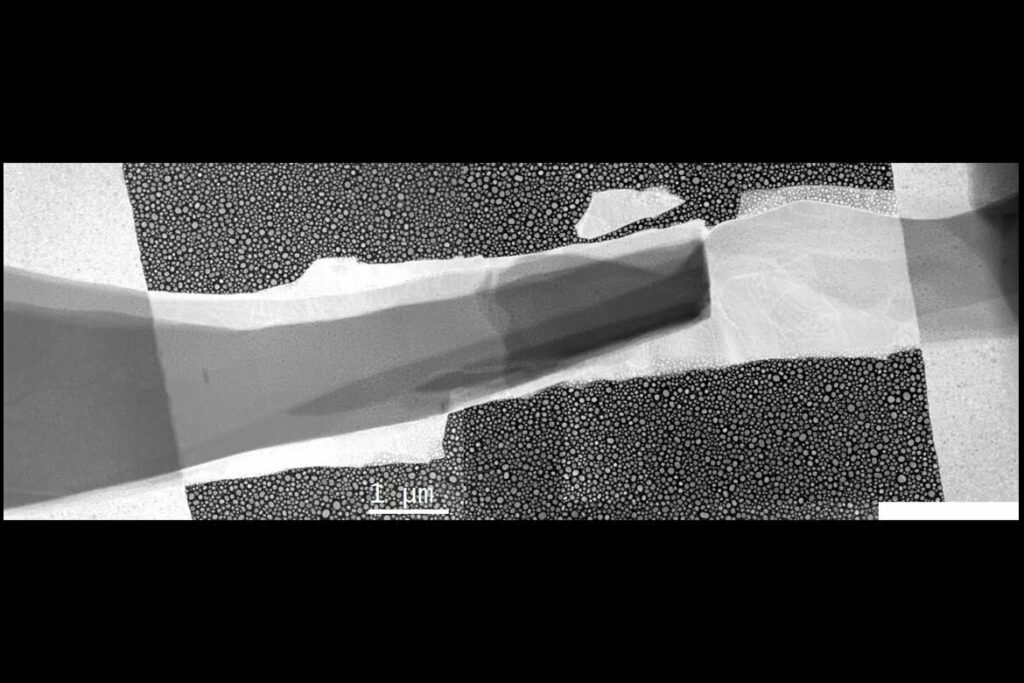
By using the same physical principles that have been powering instruments aboard NASA’s Voyager spacecraft for the past 40 years, researchers at UCLA have devised the smallest refrigerator in the world. The thermoelectric cooler is only 100 nanometers thick — roughly 500 times thinner than the width of a strand of human hair — and could someday revolutionize how we keep microelectronics from overheating.
“We have made the world’s smallest refrigerator,” said Chris Regan, who is a UCLA physics professor and lead author of the new study published this week in the journal ACS Nano.
Instead of your vapor-compression system inside your refrigerator, the tiny device developed by Regan’s team of researchers is thermoelectric. When two different semiconductors are sandwiched between metal plates, two things can happen.
If heat is applied, one side becomes hot, while the other remains cool. This temperature difference can be harvested to generate electricity. Case in point, the Voyager spacecraft, which is believed to have traveled beyond the limits of the solar system after it visited the outermost planets in the 1970s, is still powered to this day by thermoelectric devices that generate electricity from heat produced by a plutonium nuclear reactor.
This process also works in reverse. When electricity is applied, one semiconductor heats up, while the other stays cold. The cold side can thus function as a cooler or refrigerator.
What the UCLA physicists were able to do is scale down thermoelectric cooling by a factor of more than 10,000 compared to the previous smallest thermoelectric cooler.
They did so using two standard semiconductor materials: bismuth telluride and antimony-bismuth telluride. Although the materials are common, the combination of the two bismuth compounds in two-dimensional structures proved to be excellent.
Typically, the materials employed in thermoelectric coolers are good electrical conductors but poor thermal conductors. These properties are generally mutually exclusive — but not in the case of the atom-thick bismuth combo.
“Its small size makes it millions of times faster than a fridge that has a volume of a millimeter cubed, and that would already be millions of times faster than the fridge you have in your kitchen,” Regan said.
“Once we understand how thermoelectric coolers work at the atomic and near-atomic level,” he said, “we can scale up to the macroscale, where the big payoff is.”
One of the biggest challenges the researchers had to face was measuring the temperature at such a tiny scale. Your typical thermometer simply won’t do. Instead, the physicists employed a technique that they invented in 2015 called PEET, or plasmon energy expansion thermometry. The method determines temperature at the nanoscale by measuring changes in density with a transmission electron microscope.
In this specific case, the researchers placed nanoparticles of indium in the vicinity of the thermoelectric cooler. As the device cooled or heated, the indium correspondingly contracted or expanded. By measuring the density of indium, the temperature of the nano-cooler could be determined precisely.
“PEET has the spatial resolution to map thermal gradients at the few-nanometer scale—an almost unexplored regime for nanostructured thermoelectric materials,” said Regan.
The winning combination of semiconductors found by the UCLA physicists may one day be brought to the macro scale, enabling a new class of cooling devices with no moving parts that regulate temperature in telescopes, microelectronic devices, and other high-end devices.