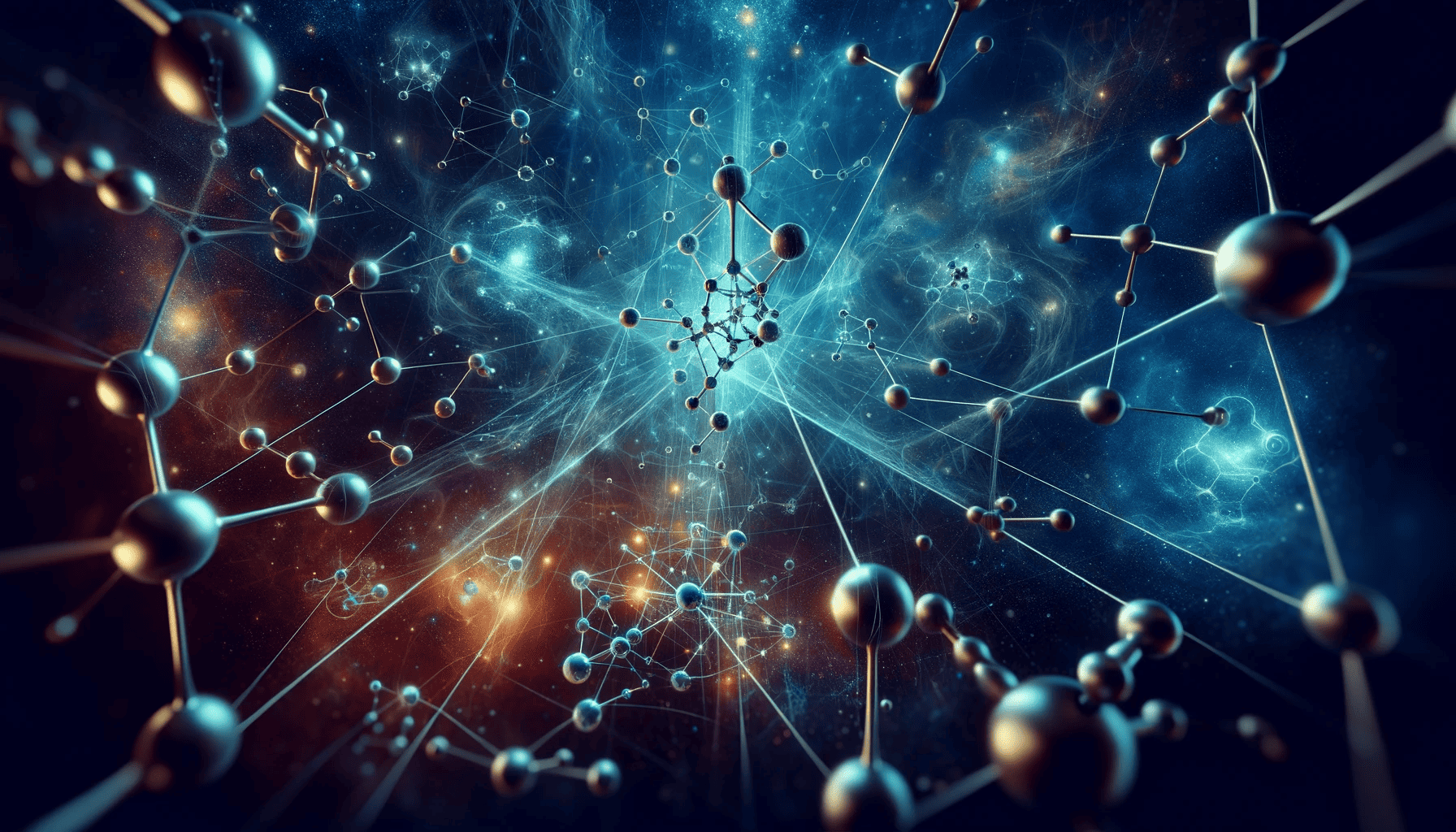
- 🔬 Princeton physicists entangle molecules, extending quantum mechanics boundaries.
- đź’» Entangled molecules could be key to future quantum computers and advanced sensors.
- đź‘» The findings demonstrate “spooky action at a distance” also works for molecules, not just atoms and ions.
Princeton physicists have successfully linked individual molecules into quantum mechanically entangled states. This groundbreaking feat allows molecules to remain interconnected across vast distances, a phenomenon often described as one of the most bizarre in quantum mechanics.
New possibilities in quantum science
This linkage persists regardless of the physical distance between the molecules, embodying the concept of “spooky action at a distance” as once skeptically noted by Albert Einstein. Previously, only individual atoms and ions could be coaxed into this state.
The research, led by Assistant Professor Lawrence Cheuk and his team at Princeton University, marks a pivotal advancement in understanding quantum entanglement. Entanglement, a core principle of quantum mechanics, occurs when particles become so deeply connected that the state of one instantaneously influences the other, no matter the distance separating them.
Cheuk and his team envision entangled molecules as foundational elements for future technologies like quantum computers, capable of outperforming traditional computing in specific tasks. Additionally, quantum simulators and sensors, leveraging entanglement, promise advancements in modeling complex materials and enhanced measurement capabilities, respectively.
Computing devices based on quantum phenomena like entanglement are supposed to be orders of magnitude more powerful than conventional computers based on silicon transistors. Their edge or “quantum advantage” stems from the principles of superposition and quantum entanglement, where quantum bits, or qubits, can exist in multiple states simultaneously, unlike the binary states of classical computer bits.
However, achieving controllable quantum entanglement remains extremely challenging. Qubits are highly sensitive to noise and hold their quantum state typically for very short periods before losing ‘coherence’. As a result, the current state of the art is crippled by errors and today’s quantum computers are unlikely to output correct answers even for relatively trivial programs — for now.
This explains why the quantum computing landscape is rich in dozens of competing technologies. There are quantum computers that work with trapped ions, photons, and superconducting circuits — just to name a few — all vying for the billion-dollar breakthrough that might finally fulfill the industry’s promise of taking computers to the next level.
Now, with this most recent advance, quantum computers that use molecular qubits can be added to this growing list of experimental technologies.
“What this means, in practical terms, is that there are new ways of storing and processing quantum information,” said Yukai Lu, a graduate student in electrical and computer engineering and a co-author of the paper.
“For example, a molecule can vibrate and rotate in multiple modes. So, you can use two of these modes to encode a qubit. If the molecular species is polar, two molecules can interact even when spatially separated.”
Despite being notoriously difficult to control due to their complexity, Cheuk and colleagues have shown molecules are promising candidates. In their experiment, the scientists used a sophisticated “tweezer array” whereby a system of tightly focused laser beams manipulated individual calcium monofluoride molecules.
The laser system cooled the molecules to temperatures a fraction of a degree above absolute zero. At such an ungodly low temperature, vibration is almost nonexistent, making the molecules almost perfectly still. Pairs of calcium monofluoride were ultimately coaxed to enter a quantum entanglement state by correlating their dipolar interaction.
Reinforcing their findings, a separate group at Harvard University and MIT achieved similar results, validating the reliability and potential of molecular tweezer arrays in quantum science.
“The fact that they got the same results verify the reliability of our results,” Cheuk said in a press release. “They also show that molecular tweezer arrays are becoming an exciting new platform for quantum science.”
As we stand at the brink of a new era in quantum science, the implications of these discoveries are profound. From reshaping computing to redefining how we understand the fabric of our universe, the entanglement of molecules opens a world of possibilities, beckoning a future where quantum mechanics moves from theoretical wonder to practical reality.
The findings appeared in the journal Science.