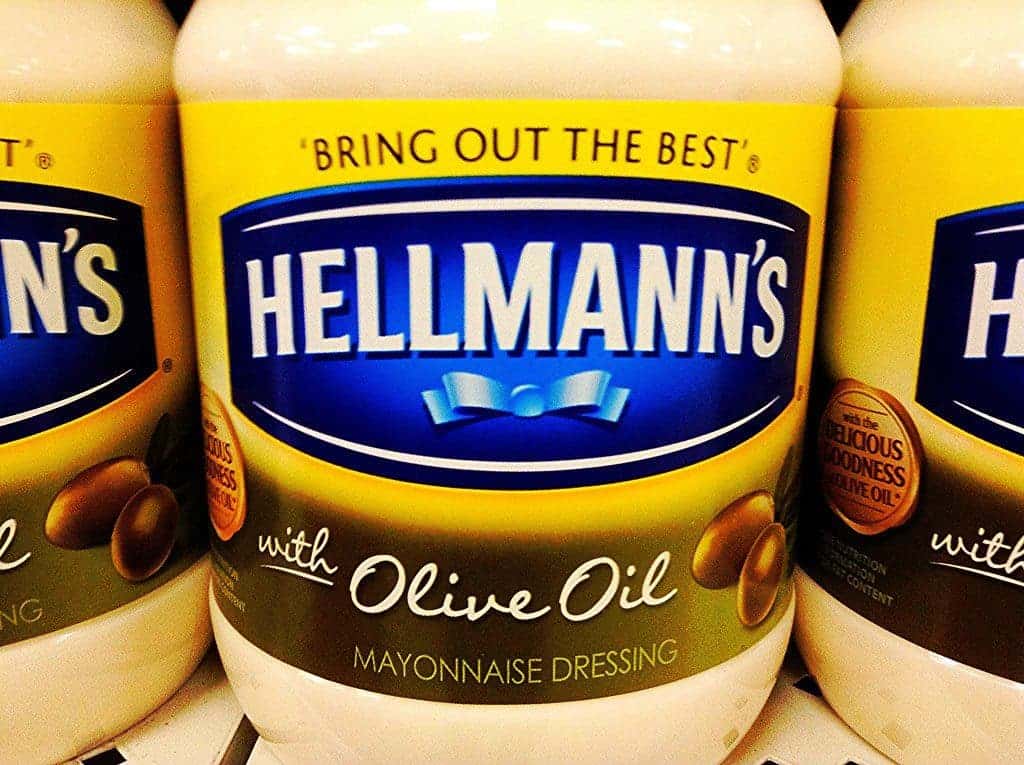
Physicists at Lehigh University have used a common household item to study the fundamental hydrodynamics inside one of the most promising types of fusion reactors. The experiments involving mayonnaise gave the scientists new insights into what may be happening when gas and molten metal mix under the influence of high acceleration and centrifugal force.
Who knew that mayo has such an important role to play in a technology that might recreate the power of the sun here on Earth?
Fusing mayo
Nuclear fusion occurs when two smaller atoms collide at very high energies to merge, creating a larger, heavier atom. This is the nuclear process that powers the sun’s core, which in turn drives life on Earth. One promising method for achieving fusion that scientists in the United States are currently exploring is called inertial confinement. At research facilities such as the Lawrence Livermore National Laboratory and Los Alamos National Laboratory, scientists confine a gas — usually hydrogen isotopes — by freezing it inside pea-sized metal pellets. The pellets are placed inside a chamber where they are hit by a high-powered laser that can generate up to a few million Kelvin (400 million degrees Fahrenheit) — conditions ripe for fusion.
The extreme heat causes the gas inside to expand, bursting the metal casing before fusion can be reached. The process is similar to a balloon being squeezed — at some point, the rubber balloon bursts under pressure from the air inside.
In order to produce fusion in inertial confinement, scientists first need to solve the problem of the molten metal and heated gas mix — and this is where mayo comes in. The material properties of the mix at high temperatures are similar to that of mayo at low-temperature, according to Arindam Banerjee, an associate professor of mechanical engineering and mechanics at Lehigh University.
Banerjee’s area of expertise is in Rayleigh-Taylor instability, a phenomenon which occurs between materials of different densities when the density and pressure gradients are in opposite directions creating an unstable stratification.
“In the presence of gravity—or any accelerating field—the two materials penetrate one another like ‘fingers,'” Banerjee said in a statement.
Investigating this kind of instability is extremely challenging because it happens almost in an instant and the large measurement uncertainties of accelerated solids.
For their study, Banerjee and colleagues poured Hellman’s Real Mayonnaise into a Plexiglass container and then accelerated the sample inside a rotating wheel. The progress of the material was tracked with a 500fps high-speed camera, whose images were fed into an image processing algorithm that could detect parameters associated with Rayleigh-Taylor instability. The wavelength and amplitude growth rates were finally compared to existing analytical models.
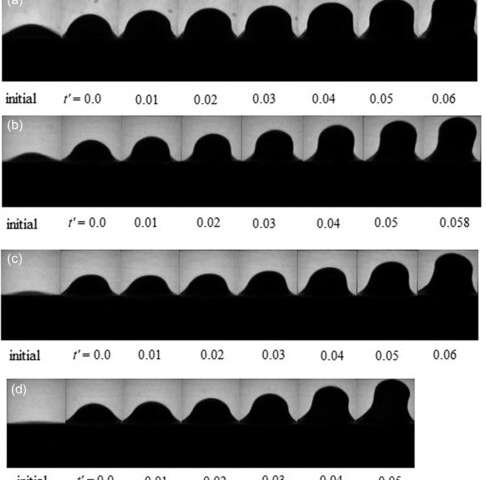
These experiments allowed the research team to visualize both the elastic-plastic and instability evolution of the material. The authors concluded that the onset of the instability (“instability threshold”) was related to the size of the amplitude (perturbation) and wavelength (distance between crests of a wave) applied.
“There has been an ongoing debate in the scientific community about whether instability growth is a function of the initial conditions or a more local catastrophic process,” says Banerjee. “Our experiments confirm the former conclusion: that interface growth is strongly dependent on the choice of initial conditions, such as amplitude and wavelength.”
In the future, these findings will help researchers design better conditions for inertial confinement. Step by step, little by little, the world is moving closer to achieving nuclear fusion. On that note, researchers have triggered fusion before — it’s just that the energy required to trigger the reaction was larger than the energy produced by it. Some of the most promising fusion reactors include the International Thermonuclear Experimental Reactor (ITER) in France and the Wendelstein 7-X reactor in Germany.
The findings appeared in the journal Physical Review E.