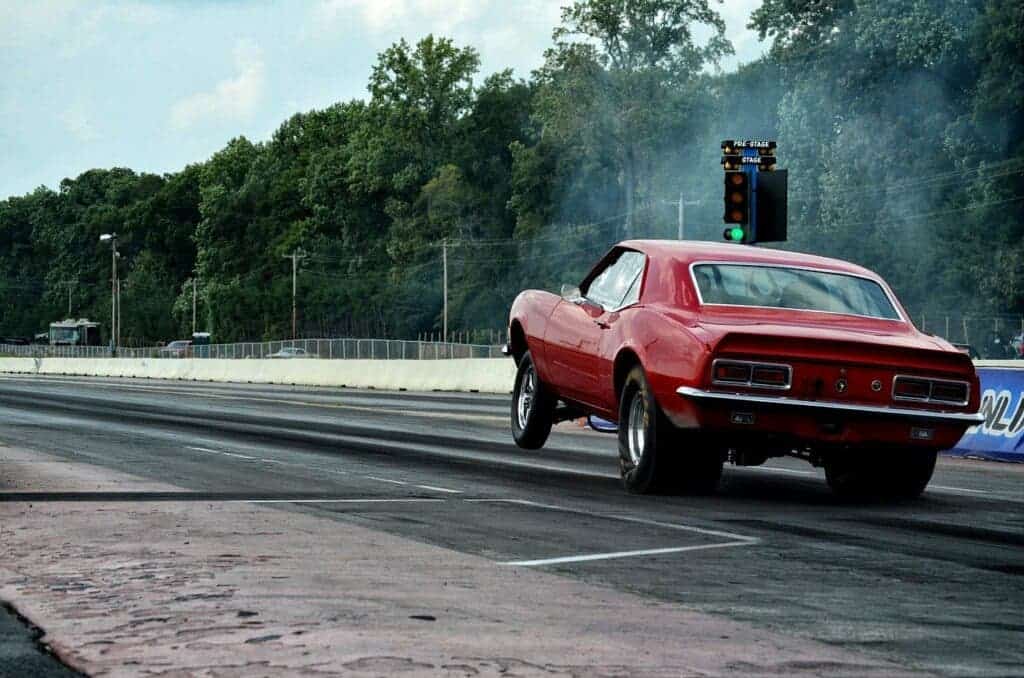
A cheap and readily available polycrystalline material may finally open the door for commercial thermoelectric generators that convert waste heat into electricity. However, other challenges have to be solved before you can put your car’s engine and tailpipe to work.
Humans generate a lot of waste, and energy is no exception
When a power plant burns coal or an internal combustion engine vehicle ignites gasoline, most of the fuel’s chemical energy isn’t converted into useful electricity or work — it’s turned into heat. A typical American power plant, for instance, only converts 30% of the coal’s energy into net electricity. And, in a sense, your car is, first and foremost, a furnace on wheels.
It’s no wonder that scientists have sought to minimize this massive heat loss to the best of their abilities. Some of this otherwise wasted energy can be used for heating during the cold season, for instance. Alternatively, the waste heat can be used to co-generate electricity in a power plant by heating water to make steam that turns turbines. But ideally, you’d want to directly convert the heat (uncoordinated motion of the particles in a material) into the more versatile electricity (controlled movement wave of electrons). This is where thermoelectricity comes in.
The thermoelectric effect arises when you have two different semiconductors sandwiched between metal plates and one side is hot, while the other is cold. A thermoelectric generator can harvest the energy from this temperature difference and turn it into electricity.
The Voyager spacecraft, which is believed to have traveled beyond the limits of the solar system after it visited the outermost planets in the 1970s, is still powered to this day by thermoelectric devices that generate electricity from heat produced by a plutonium nuclear reactor. The same energy powers the Curiosity and Perseverance rovers operated by NASA on Mars.
This process also works in reverse. When electricity is applied, one semiconductor heats up, while the other stays cold. The cold side can thus function as a cooler or refrigerator.
The problem is that these thermoelectric generators are either extremely inefficient or expensive, which has reserved them for very niche applications like aerospace. Additionally, the materials involved tend to contain toxic elements.
When two materials of different temperatures are in contact or close enough, they will eventually reach the same temperature. When this happens, there is no more thermoelectric effect. Designing semiconductors that have poor thermal conductivity but nevertheless carry electricity is no trivial engineering challenge. This makes recent developments by physicists at Seoul National University and Northwestern University all the more exciting.
It all started in 2016 when a team of researchers at Northwestern led by materials scientist Mercouri Kanatzidis devised a thermoelectric generator using tin and selenium powders, two readily available and cheap materials. The powders were processed into grains of polycrystalline tin selenide that can be compressed into thin ingots no larger than 3 centimeters in length, making them ideal for devices.
Kanatzidis expected the boundaries between the grains to slow down heat transfer. However, when the researchers tested the material, they found that thermal conductivity was extremely high, making for a very poor thermoelectric generator.
They eventually discovered the problem: an ultrathin film of tin oxide had formed around the polycrystalline grains before they had the chance to be pressed into ingots. This film allows heat to transfer very quickly from grain to grain.
In their new study published this week in Nature Materials, Kanatzidis and colleagues presented a new method that expels any oxygen from the tin and selenium precursors, leaving behind just pure polycrystalline tin selenide.
The method proved successful, raising the thermoelectric efficiency of the polycrystalline material almost threefold. However, a fully working thermoelectric device will require additional innovation.
The tin selenide material conducts only positive charges. To complete a circuit you also need an n-type antagonist that conducts negative charges. Luckily, an n-type single-crystal tin selenide counterpart was recently demonstrated by Chinese scientists at Beihang University.
If the two prove a match, then commercial applications for wide-scale adoption of thermoelectric generators may be just around the corner.