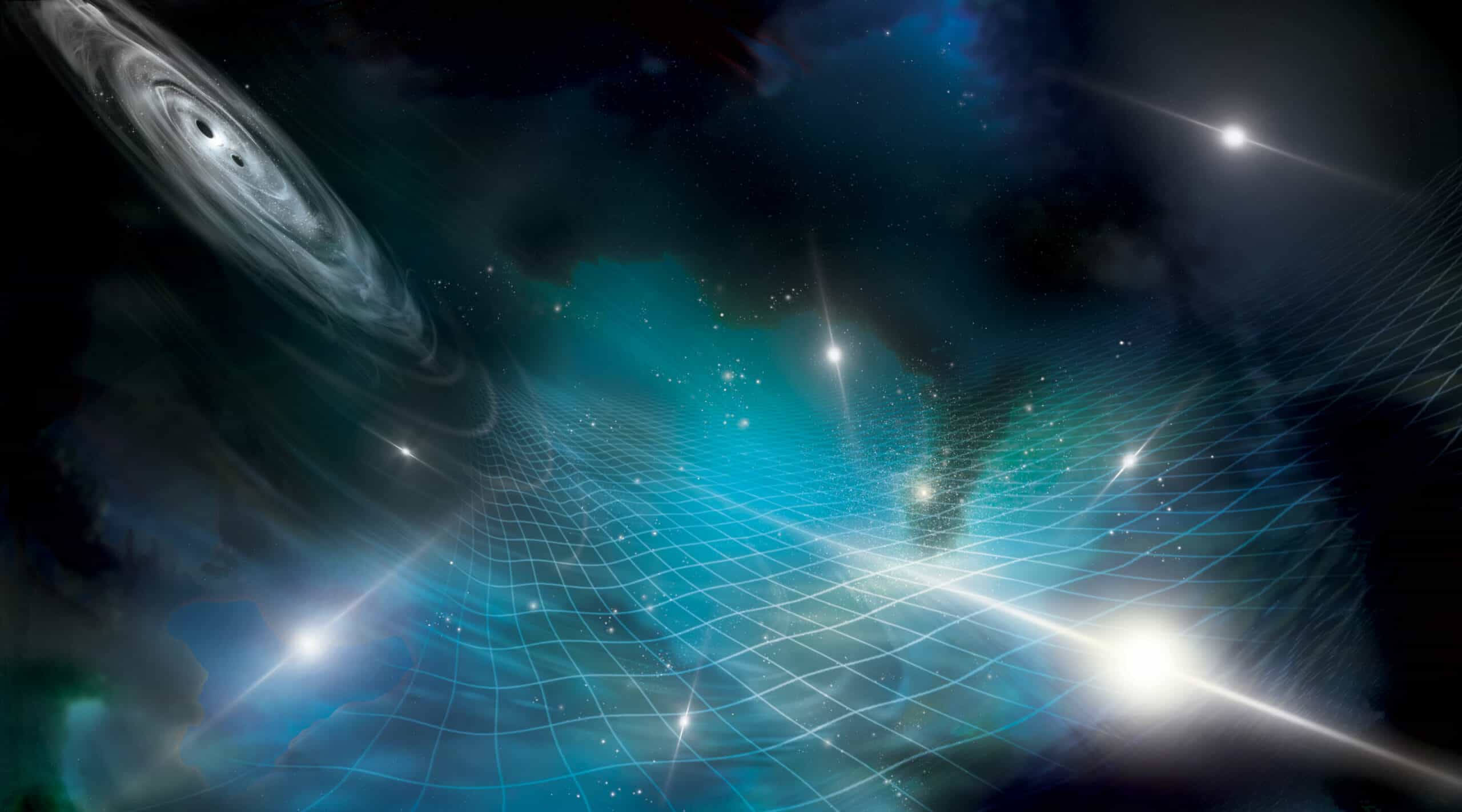
Following 15 years of data collection in a galaxy-sized experiment, scientists have “heard” the perpetual chorus of gravitational waves rippling through our universe for the first time—and it’s louder than expected.
These waves all hum and resonate together, creating a kind of cosmic noise known as the gravitational wave background, which has long been theorized but never detected before. These waves permeate the universe, constantly surfing the fabric of spacetime around us. They are literally everywhere — and we now have the confirmation.
These ultra-low-frequency gravitational waves hold the potential to unlock some of the Universe’s greatest enigmas. By investigating these waves, scientists hope to gain a deeper understanding of the formation and behavior of supermassive black holes in binary systems, as well as events that transpired during the infancy of our Universe.
“The results presented today mark the beginning of a new era in our exploration of the Universe, as we unveil some of its mysteries. Our analysis reveals a consistent signal observed across all the pulsars in the array, suggesting that these cosmic clocks are being swayed by the waves of spacetime,” said Professor Alberto Vecchio, from the University of Birmingham’s Institute for Gravitational Wave Astronomy.
“It’s really the first time that we have evidence of just this large-scale motion of everything in the universe,” said Maura McLaughlin, co-director of NANOGrav, the research collaboration that published the results in The Astrophysical Journal Letters.
What is the gravitational wave background?
Gravitational waves are disturbances in the fabric of spacetime that propagate outward from their source at the speed of light. They are produced by the acceleration or motion of massive objects, such as black holes, neutron stars, or even the merging of two such entities. Just as a stone creates ripples in a pond when it is thrown, massive objects create ripples in spacetime as they move, generating gravitational waves.
The discovery of the existence of gravitational waves is a direct consequence of Einstein’s general theory of relativity, which he formulated in 1915. In this groundbreaking theory, Einstein proposed a new understanding of gravity as the curvature of spacetime caused by mass and energy. According to general relativity, massive objects, like stars and planets, create a curvature in spacetime around them, which influences the motion of other objects nearby. But this curvature can manifest itself as gravitational waves when these objects are accelerated or disturbed.
Einstein himself was initially skeptical about the existence of gravitational waves, as they seemed to be incredibly subtle and difficult to detect. However, he mathematically predicted their existence and considered them an essential consequence of his theory.
It wasn’t until a century later, in 2015, that the Laser Interferometer Gravitational-Wave Observatory (LIGO) made the groundbreaking discovery of the first direct evidence of gravitational waves.
However, these newly identified cosmic ripples, known as gravitational waves are quite different. They carry a staggering amount of energy—roughly a million times more than the gravitational wave bursts from the black hole and neutron star mergers previously detected by Nobel Prize-winning experiments like LIGO and Virgo.
And because this background ‘hum’ operates at a much lower frequency, the detection methods involved were strikingly different.
The LIGO detectors are enormous L-shaped interferometers with two perpendicular arms, each measuring about 4 kilometers (2.5 miles) in length. They are located in Livingston, Louisiana, and Hanford, Washington. The basic principle behind the operation of these interferometers involves measuring the minute changes in the relative lengths of the two arms caused by passing gravitational waves.
However, in order to detect the faint hum of background gravitational waves, scientists got really creative — and had to wait a lot of time.
Keeping time with dead stars
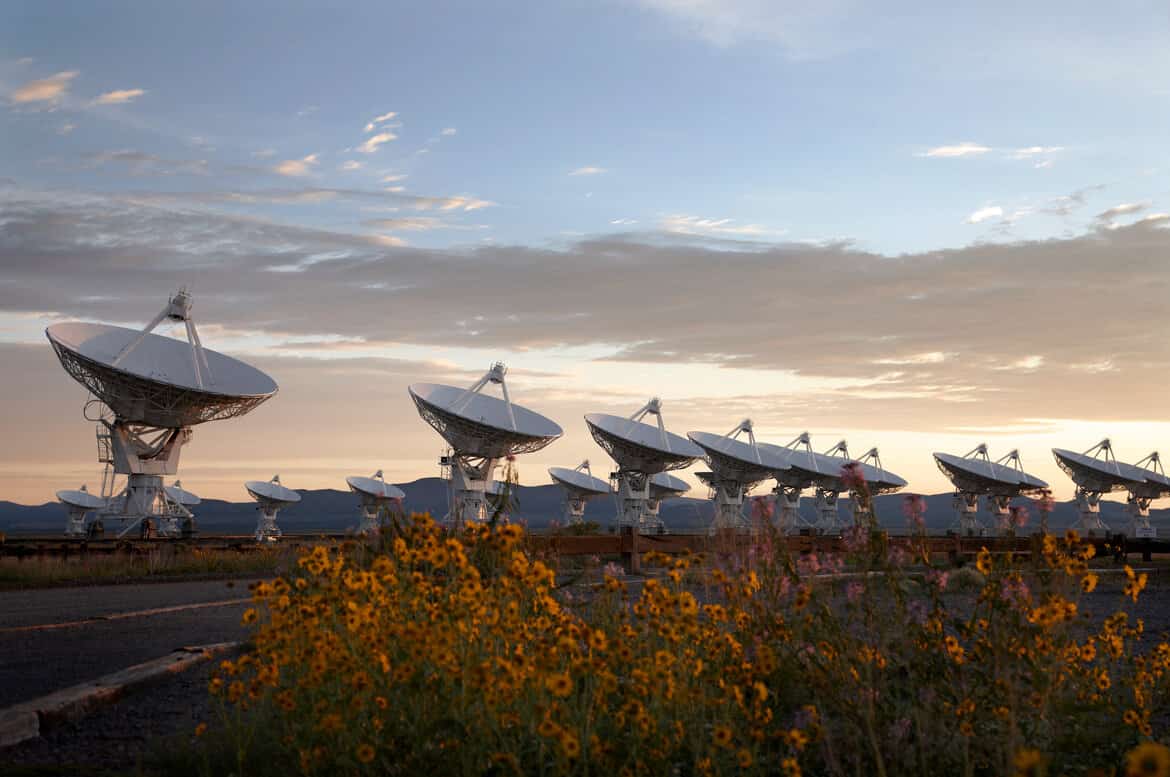
To catch the elusive fluctuations caused by gravitational waves, scientists employ pulsar timing arrays, using multiple radio telescopes to observe numerous pulsars over extended periods.
Pulsars are dense remnants left behind after the explosive demise of massive stars, known as supernovae. What makes them truly remarkable is their extraordinary behavior—emitting regular beams of radiation that sweep across the cosmos with astonishing precision. Imagine them as cosmic lighthouses that regularly light up from your point of view and then dim with predictable timing.
These arrays act as cosmic timekeepers, with the stopwatch-like regularity of pulsars serving as a valuable tool. If gravitational waves cause space-time to expand and contract, then they should also affect these pulsar radio flashes.
By harnessing the collective power of 25 pulsars, scientists fashioned a colossal gravitational-wave detector, stretching across galaxies. As pulsar radio waves traverse the vast expanse of space and time, gravitational waves weave their influence. These irregularities change the incoming information that we detect.
By comparing the time delays of many pairs of pulsars over an observation period spanning 15 years, astronomers could decipher the telltale signs of ultra-low frequency gravitational waves.
Four teams, each based in Europe, India, Australia and China, have presented their findings at the same time, in a coordinated release of data. This is notable in itself because there is a great incentive to be the first to ‘break the news’ for high-impact scientific discoveries. By releasing all the papers at the same time, the researchers demonstrated fantastic diplomacy and highlighted the interdisciplinary nature of this challenging work. It’s not about a single person getting all the laurels, but rather recognizing that this is truly a team effort.
Among them, NANOGrav stands as the most confident in their results. They collected data over 15 years using radio telescopes such as the Green Bank Telescope in West Virginia and the now-collapsed Arecibo Observatory in Puerto Rico. These waves exhibit oscillations with periods ranging from one to ten years, providing a glimpse into the cosmic rhythm.
The rise of a new era
It’s quite mind-boggling when you realize that these scientists actually hacked the entire galaxy to act as a giant gravitational wave detector.
Although the results presented today do not meet the stringent gold standard of scientific discovery — which requires a probability of less than one in a million chance occurrence — these findings are nevertheless groundbreaking. Efforts are underway to combine data sets from the European Pulsar Timing Array, the Indian Pulsar Timing Array, the PPTA, and NANOGrav, under the banner of the International Pulsar Timing Array.
This collaborative endeavor aims to expand the array to encompass over 100 pulsars, potentially leading to more definitive confirmation of ultra-low frequency gravitational waves.
The implications can’t be understated. Gravitational waves carry information about the objects and events that generate them. By analyzing the properties of these waves, scientists can decipher the masses, spins, and orbital dynamics of the celestial objects that produced them, contributing to our understanding of the universe’s composition and evolution. This includes the merger and collision of supermassive black holes or even entire galaxies.
A new era in astronomy is upon us.
- The NANOGrav 15-year Data Set: Evidence for a Gravitational-Wave Background, The Astrophysical Journal Letters (2023). DOI: 10.3847/2041-8213/acdac6
- Gabriella Agazie et al, The NANOGrav 15 yr Data Set: Observations and Timing of 68 Millisecond Pulsars, The Astrophysical Journal Letters (2023). DOI: 10.3847/2041-8213/acda9a
- Gabriella Agazie et al, The NANOGrav 15 yr Data Set: Detector Characterization and Noise Budget, The Astrophysical Journal Letters (2023). DOI: 10.3847/2041-8213/acda88
- Adeela Afzal et al, The NANOGrav 15 yr Data Set: Search for Signals from New Physics, The Astrophysical Journal Letters (2023). DOI: 10.3847/2041-8213/acdc91
- Astrophysical Interpretation of a Gravitational Wave Background from Massive Black Hold Binaries (accepted for publication in ApJL)
- Bayesian Limits on GWs from Individual SMBHBs (accepted for publication in ApJL)