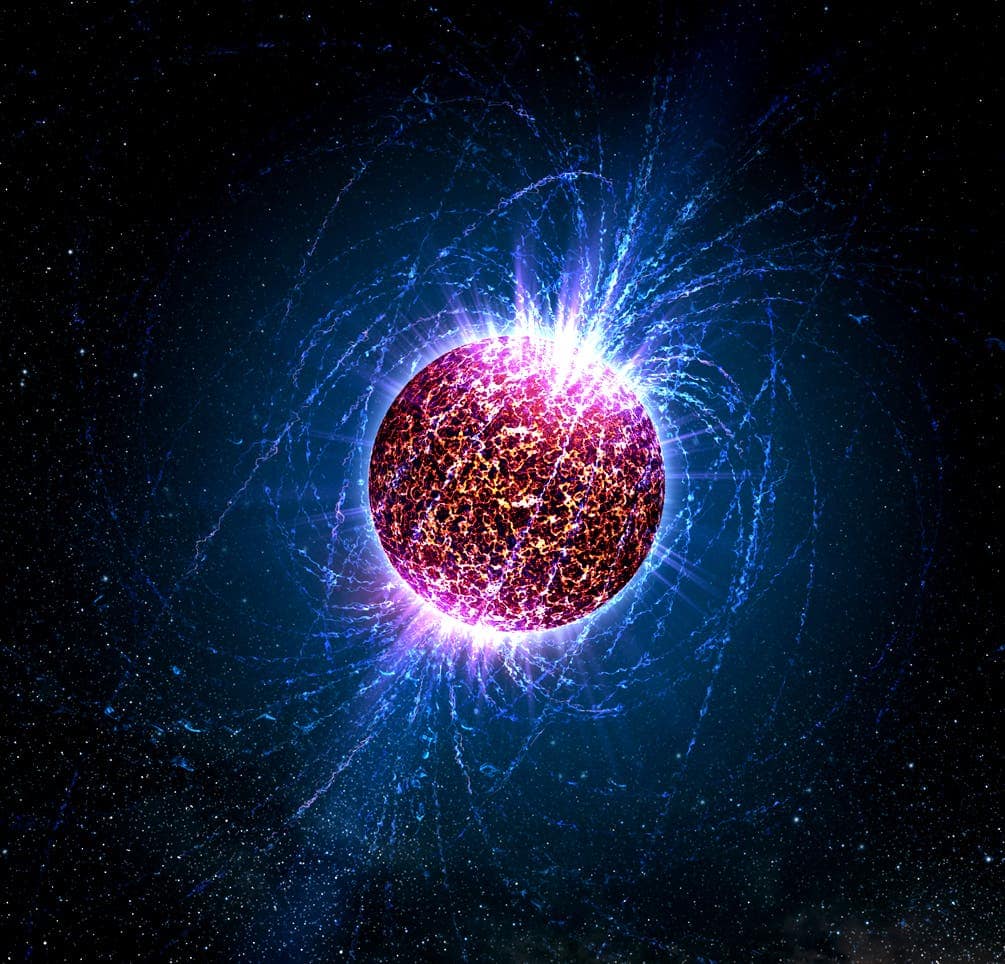
Neutron stars are the densest matter that should “normally” exist (black holes are denser, but they’re really weird, so let’s not count those for now). As the traditional example goes, a single teaspoon of neutron star matter weighs around as much as the entire human race put together — about 100 million tons.
All the matter we see around us seems very dense, but at an atomic level, there’s a lot of empty space. The reason why neutron stars are so dense is that they are composed of closely packed neutrons. If you get rid of all the electrons and only keep the neutrons, you can do that — but you need very special conditions.
A neutron star is the collapsed core of a giant star which (before collapsing) has a mass of around 10-29 solar masses. Astronomers have studied these objects for decades, but many things about them are still not clear. For instance, what really happens to the neutrons — do they become a sort of superfluid that flows freely, or do they break down into subatomic particles? Also, where exactly is the tipping point at which a collapsing star produces a black hole versus a neutron star?
This newly-discovered star could help answer some of those questions.
“Neutron stars are as mysterious as they are fascinating,” said Thankful Cromartie, a graduate student at the University of Virginia and Grote Reber pre-doctoral fellow at the National Radio Astronomy Observatory in Charlottesville, Virginia. “These city-sized objects are essentially ginormous atomic nuclei. They are so massive that their interiors take on weird properties. Finding the maximum mass that physics and nature will allow can teach us a great deal about this otherwise inaccessible realm in astrophysics.” Cromartie carried this work as part of her PhD project.
The star, which is unceremoniously called J0740+6620, is a pulsar — a highly magnetized rotating type of neutron star that emits a beam of electromagnetic radiation. It packs a mass 2.17 times larger than that of our Sun, although it’s only 30 kilometers across. This is very close to the limit of how compact a single object can be without crushing itself into a black hole — as suggested by very recent work on gravitational waves observed from colliding neutron stars.
In order to carry out these measurements, researchers took advantage of one of the main property of pulsars: the special pulse they emit.
Pulsars are called this way because they emit two radio beams through their magnetic poles, kind of like how a spinning lighthouse emits light. Since pulsars revolve at dazzling speeds (sometimes, at 24% of the speed of light), the pulses can be used as a sort of cosmic timekeepers. But in itself, this is not enough to accurately calculate the mass of the pulsar.
There’s another property which researchers use to this end: something called the Shapiro delay.
The neutron star has a companion — a white dwarf. As the signal from the pulsars passes the white dwarf, the dwarf’s gravity is just large enough to distort the environment around it, slightly warping the space-time in its vicinity (in accordance with Einstein’s general theory of relativity). This means that the pulses have to travel just a bit more than they should without the gravitational effect — and this extra bit is indicative of the white dwarf’s gravitational pull, and therefore, its mass.
After this, once the mass of the dwarf was calculated, the mass of the pulsar can also be determined precisely through a relatively straightforward process.
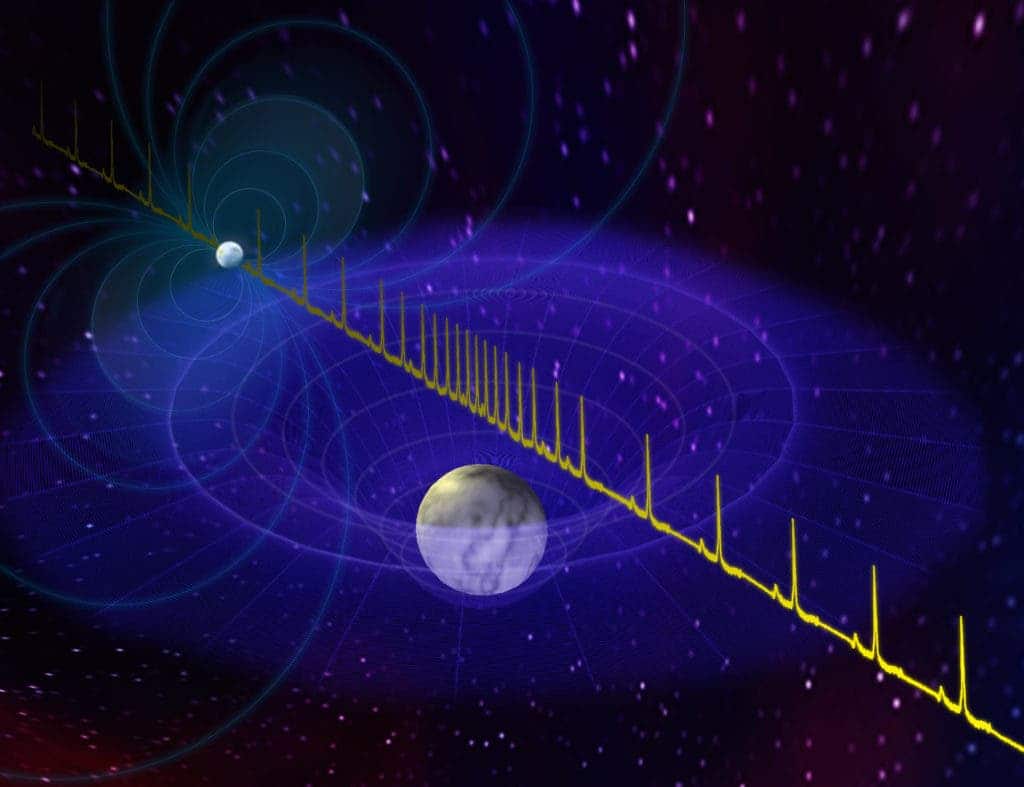
This showed that the neutron star is very close to becoming a black hole, but is not quite there.
Researchers say that this environment creates the perfect setup to measure the mass of the neutron star, acting as a cosmic lab. With every such system that they discover, astronomers get a better approximation of when stars become black holes, and when they remain neutron stars.
“The orientation of this binary star system created a fantastic cosmic laboratory,” said Scott Ransom, an astronomer at NRAO and coauthor on the paper. “Neutron stars have this tipping point where their interior densities get so extreme that the force of gravity overwhelms even the ability of neutrons to resist further collapse.
“Each ‘most massive’ neutron star we find brings us closer to identifying that tipping point and helping us to understand the physics of matter at these mindboggling densities.”
The study has been published in Nature Astronomy.