We tend to not think about it, but around 10% of the human population currently lives in the risk zone of active volcanoes. While the other 90% of us are relatively safe from the eruptions of smaller volcanoes (such as the Cumbre Vieja in La Palma, which recently erupted), if one of the larger magmatic systems were to erupt, we would all find ourselves in one hot pickle — no matter where we reside.
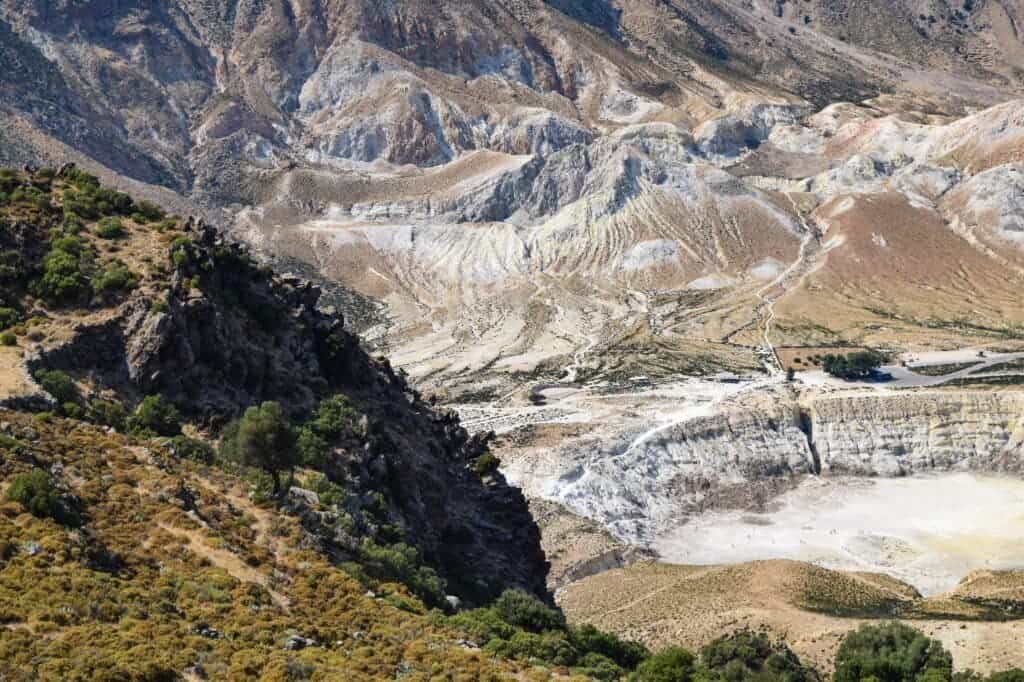
The problem is, we have plenty of those big, mean volcanoes to start with — and they’re often closer to home than you’d think. Even in Europe, where volcanic eruptions are relatively rare to begin with, there are Nisyros, Santorini, Hekla, or Campi Flegrei. We don’t even want to think about what an eruption from the likes of Yellowstone, Toba, or Tambora would bring — and yet we have to.
When volcanoes erupt
Nowadays, with the knowledge and technology we have at our disposal, it is pretty easy to know when a particular volcano is going to erupt. The rise of magma through the crust triggers swarms of small intensity earthquakes, it causes the rocks to bulge, and hot waters and gas to reach the surface well before the magma does, heralding incoming trouble.
What we don’t really know — and this has bugged volcanologists for decades — is how a volcano is going to behave during the eruption. Will it generate effusive eruptions that lead to relatively mild lava flows which can damage property but are relatively harmless to people? Or will it trigger violent explosions, which eject clouds of hot gas and ash, or even disintegrate entire volcanic structures, leaving behind caldera depressions instead of mountains?
To solve this problem, volcanologists have mostly focused on what happens in the volcanic conduit — the pipeline that connects the magma chamber to the surface. Once an eruption begins, magma ascends through the crust, generally for about 8-10 km before reaching the volcanic summit. During this ascent, what happens to the gas that bubbles in the magma is the key to how the volcano will erupt.
If, for example, the gas remains trapped in the melt and can’t escape to seep away, there’s a big chance the magma will explode. If on the other hand, the gas bubbles get to sneak out and leave the melt behind, or outgas, the explosive potential of the magma is neutralized and the volcano will likely ooze lava flows. Letting the gas escape is more or less like defusing a bomb, reducing the risk of a big explosion.
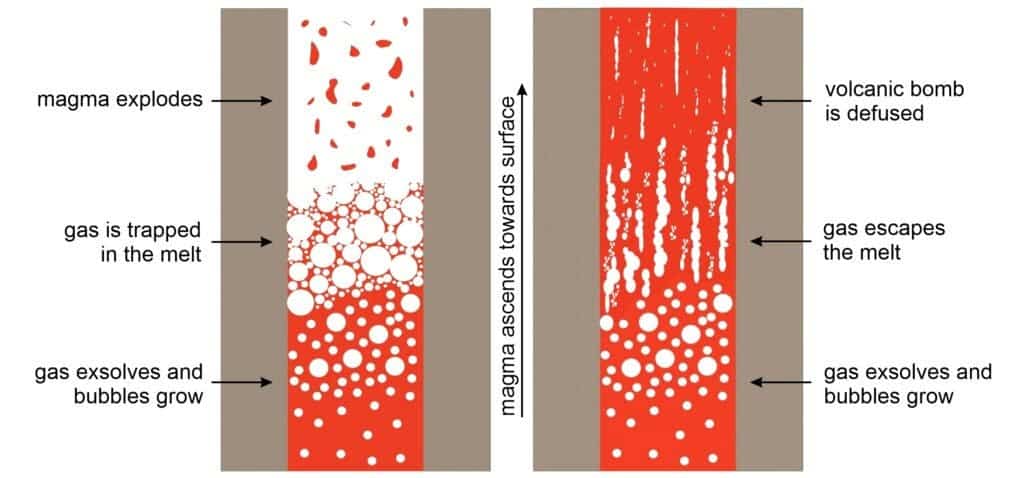
It sounds simple, but it’s deceptively complicated. Decompression, changes in ascent velocity and melt viscosity, gas bubbling and percolation, stress buildup, the mechanical resistance of the melt, and all sorts of complicated interactions between melt, crystals, gas bubbles and country rocks, will all compete or cooperate to either block the gas in the magma, or to allow it to outgas. It’s so complicated in fact that we don’t yet have a clear understanding of how all these processes interact, and we are still unable to build robust numerical models to simulate all of them. Even if we would reach the required level of understanding, and if we’d be able to forecast eruptive styles based on conduit processes, it would only give us a few minutes’ worth of time to do anything about it since the magma is already on its way up.
A few minutes isn’t exactly enough to do that much in case of an incoming explosive eruption. It would give you the chance to open that bottle of wine you’ve been saving (because you may not get another opportunity) but not much more. But what can we do if we forecast the eruptive behavior of a volcano well before the eruption is even triggered? What if instead of mere minutes, we’d have weeks, months, years, even decades to prepare?
We can now stop dreaming about it and start planning, because we are one step closer to achieving this goal.
Predicting eruptions
A recent study published in Nature Geoscience by researchers from the Swiss Federal Institute of Technology (ETH Zürich, Switzerland) and Brown University (USA), with myself as one of the authors, makes a major breakthrough in the direction of forecasting eruptive styles. The question we designed the study around was: what if the magma chamber conditions can predetermine eruptive behavior, regardless (to some extent) of what happens in the conduit?
It should be possible, after all, since the magma entering the volcanic conduit inherits all its initial properties from the magma chamber.
The big difference between magma chamber processes and conduit processes is that whatever happens in the magmatic reservoir takes place over days, months, years, even thousands or tens of thousands of years, giving us ample time to detect changes. Indeed, this new study shows a striking correlation between how the magma is stored underground, and how it ultimately behaves at the surface.
The study is based on reconstructing the magmatic storage conditions of about 245 eruptions generated by 75 volcanoes worldwide, including some really famous ones. To achieve this, we relied on the chemistry of minerals and glasses from erupted products, which are windows to processes and conditions that had happened deep underground, and which we can’t really probe directly. Using this approach, we determined the temperatures of the magmas, the amounts of solid crystals floating in the melt, the content of dissolved gas it stored, and whether some of that gas might have started exsolving (or forming gas bubbles) while still in the magma chamber.
As was expected, low amounts of dissolved gas (generally lower than 3.5 wt% water) leads to effusive outpourings of lava, while higher water contents (roughly between 4 and 5.5 wt%) favor explosive events. Interestingly, however, crystallinity (the volume of solid particles in the magma) has an important say in this as well. When more than 40% of the volume of the magma consists of crystals, the eruption becomes mild no matter the stored gas content. This happens because the solid particles form a kind of skeleton that the gas bubbles connect to, allowing them to form finger channels that act like pipes. In this way, even if the magma has enough gas content to explode, the crystals help the gas permeate the melt efficiently and defuse the volcanic bomb. At the same time, a large amount of crystals increases the bulk viscosity of the magma and its resistance to flowing. By doing so, the magma is slowed down considerably on its way to the surface (even by ten times), allowing more time for the gas to escape through the finger channels.
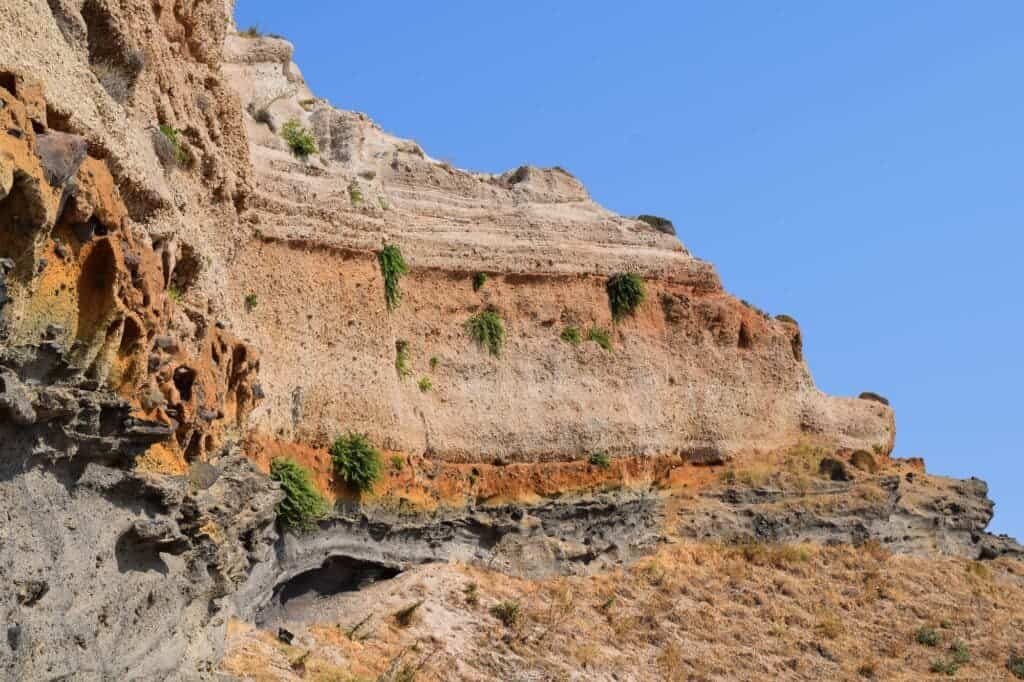
A key observation, which is counter-intuitive and bound to spark a debate in the volcanological community, is that at very high gas contents (more than 5.5 wt% water), the magmas start behaving effusively again. Why, though? The higher the gas content, the more explosive the magma should be, right? But as we found, this is not necessarily the case.
At very high dissolved gas contents, the melt is unable to store all its water in dissolved form anymore: as disseminated molecules. Instead, the molecules come together to form gas bubbles, or to exsolve. It’s very much like a stirred bottle of champagne. What we found is that magmas very rich in gas are also likely to contain quite a few gas bubbles in the magma chamber. Their presence dramatically changes how the eruption is initiated, and as a result, how it is likely to behave.
How? Well, this is where things get complicated again. Most volcanic eruptions are triggered when magma that is even hotter and comes from even greater depths, from the lower crust of the Earth, intrudes the shallow magma chamber of the volcano. Yes, for us volcanologists 10 km is shallow… This intrusion of hot magma into another body of liquid magma is known as magmatic recharge. As more magma is being crammed inside, the magmatic reservoir is being pressurized: it’s more or less like blowing a balloon that has no space to expand, while more air keeps on going inside. At some point, the balloon will just break. The same happens in a magma chamber: the rocks sealing it fail, and the hot stuff starts threading its way towards the surface.
If a magma chamber doesn’t contain gas bubbles (or contains very few of them), it pressurizes fast during magmatic recharge, and the eruption is triggered readily. When many gas bubbles are present in the magma chamber though, as the article highlights through numerical simulations, they act as a myriad of tiny cushions. Each gas bubble compresses to allow space for the extra magma that comes from below. This means that even more hot material needs to intrude the magma chamber until the surrounding rocks finally break and allow the material to erupt at the surface. More and more hot recharge coming in, and more time for it to interact with the magma chamber means that the resident melt heats up. Heating up a melt is like heating up honey: it becomes less viscous, and a less viscous melt is able to lose gas easily. See the connection?
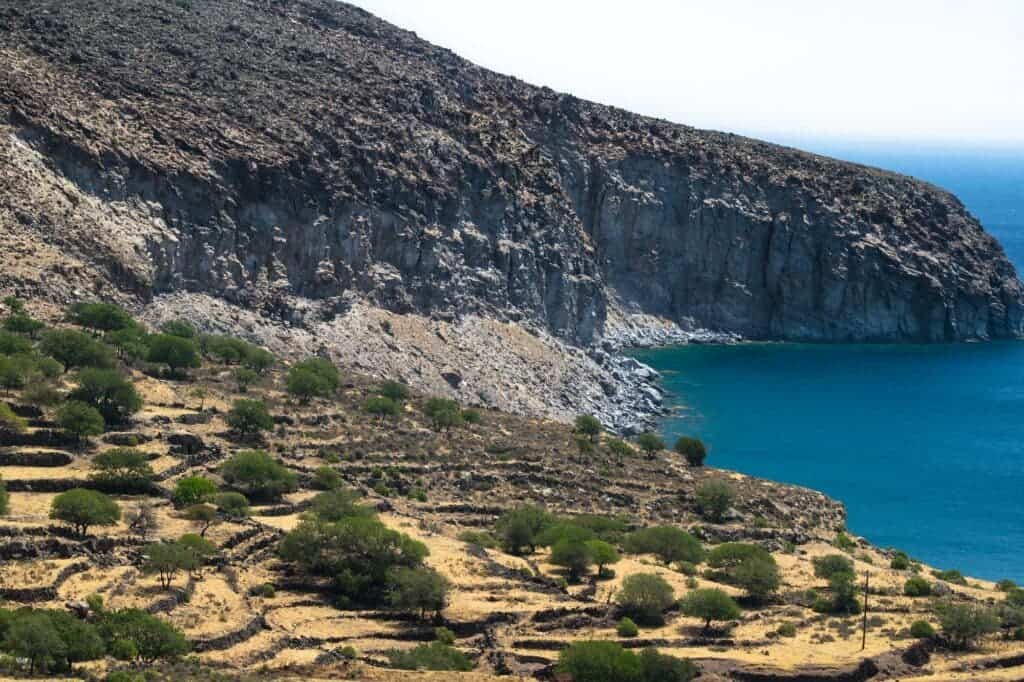
Basically, a magma chamber containing gas bubbles heats up more intensely before an eruption is initiated, it ends up feeding the conduit with a melt of lower viscosity which allows the gases to seep through it faster, and in addition it already has a multitude of gas bubbles that are ready to connect and outgas even at the base of the volcanic conduit.
In conclusion, what the article shows is a clear window of explosivity, at between 4-5.5 wt% water and at low to moderate crystallinities. All we need to do now is to find a way of looking inside active magma chambers and evaluate their state. Scanning something buried at a depth of about 8-10 km might sound science-fiction, but geophysics is here to do the job. One method, in particular, magnetotellurics, which uses the natural magnetic and electric fields of the earth, is capable to reconstruct the electrical resistivity structure of active magma chambers. By approaching the problem interdisciplinary and integrating the geophysical and volcanological data, we can use this electrical resistivity structure to estimate the crystallinity of the magmatic reservoir and to check whether significant volumes of gas bubbles are currently present or not in the magma chamber. These are two of the three key parameters required for the timely forecasting of the eruptive behaviour of volcanoes.
The study has been published in Nature Geoscience. Journal Reference:
Popa, RG., Bachmann, O. & Huber, C. Explosive or effusive style of volcanic eruption determined by magma storage conditions. Nat. Geosci. 14, 781–786 (2021). https://doi.org/10.1038/s41561-021-00827-9