Cooking started out as a very practical matter — it made food tastier, easier to chew, overall better. It’s no less practical today than it was back in the stone age, but over time it has also taken on deep cultural, social, and personal meanings throughout the world. Food can bridge different cultures and offers a context for creating or strengthening family and social ties. We compete to show off our cooking and eating skills. We celebrate special occasions with baked goods. Even most religions deal in some way or another with food.c
In other words, cooking is an integral part of the human experience, with a much wider scope than simply calming a rumbling belly. So let’s take a look at the backstage of cooking and get to know the chemical reactions that underpin fine dining.
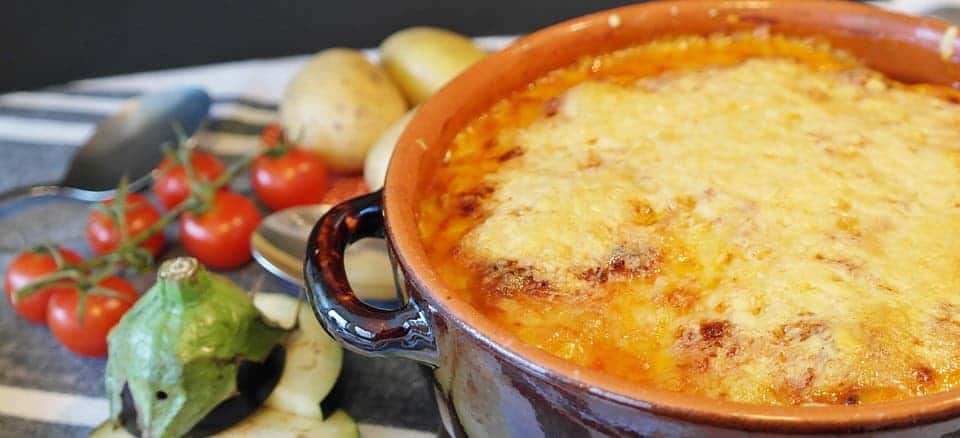
What’s cooking
Before going any further, let’s get an idea of what it actually is. The term describes any process used to prepare food for eating. These can range quite widely. Methods such as ceviche rely solely on plant acids to cook proteins. Pickling uses bacterial fermentation to preserve and prepare foodstuffs.
Different cooking methods were historically how people adapted to the rigors of their environments, maximizing the effective number of calories they could obtain while bypassing limiting factors. Ceviche, for example, offers an alternative in areas where fuel for fires is scarce. Pickling would serve to keep certain items (vegetables, for example) fresh much longer than otherwise possible, so people could stock up on food to last them during trying times, such as during winter.
However, cooking usually involves the application of heat in one way or another to prepare food. For the sake of simplicity, today we’ll be talking about this last kind of cooking.
Carbohydrates

Chemically speaking, the oft-dreaded carbs are molecules containing carbon, oxygen, and hydrogen, typically with oxygen to hydrogen ratio of 2:1. This is the same ratio as water, which will be important later on. They’re basically sugars and starches — which are also sugars, but with longer molecules. Sucrose (table sugar) is one such carb.
If you’ve ever heated table sugar, you’ve noticed it will begin to brown, then liquify, and finally bubble. The bubbling occurs due to hydrogen and oxygen breaking off from the sugars, forming water molecules, and evaporating. The browning is owed to the polymerization of caramelans (C24H36O18), caramelens (C36H50O25), and caramelins (C125H188O80). Caramel’s unique aroma is given off by volatile substances released during their pyrolysis, such as diacetyl.
So far so good, but why is caramelization important? Well, the process is key in cooking many plant-based foodstuffs. Vegetables cooked on high heat, in a stir-fry for example, will progressively brown as their starches and sugars break down and caramelize. Thankfully for us all, the volatiles released also give these veggies newfound ‘yum’. Caramelization is also partly responsible for the golden-brown hue bakers’ goods take on in the oven.
The Maillard reaction, a chemical reaction between amino acids and reducing sugars first described by French chemist Louis-Camille Maillard in 1912, is the other reaction that plays a role in baked goods‘ aroma, flavor, and golden hue. Like caramelization, it’s also a type of heat-powered browning. However, it takes place at lower temperatures (at about 140 to 165 °C / 280 to 330 °F) and creates a wide-ranging cocktail of substances.
Lipids
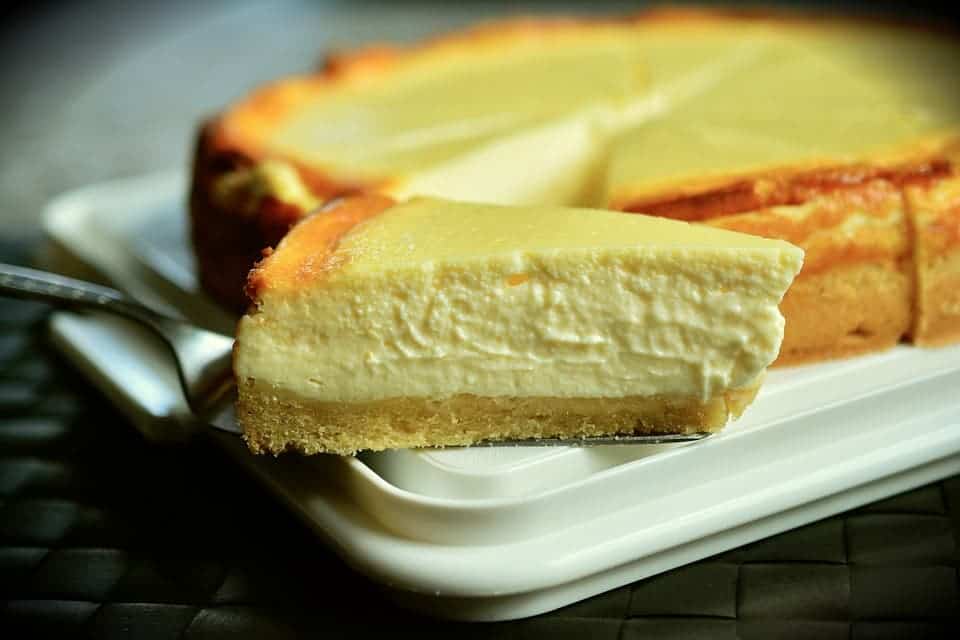
Colloquially known as fats, they are long-chain hydrocarbons. Their exact chemical nuances are a bit complex, but suffice to say that the overwhelming majority of dietary fats are triglycerides. The longer these chains get, the more they will tend to jumble up. Because of this, at room temperature fats generally tend to be solids. Obviously, there are exceptions to this rule. Oils, for example, are made up of short-chained, mostly unsaturated (we’ll get to that in a moment) fats. Apply heat, however, and fat melts — heat is energy, and enough energy allows fat’s molecular chains to shake away from and start sliding past each-other, i.e. to flow.
Fats are one of the densest energy stores organisms produce. Because of their high energetic value, your brain makes fatty food taste good so you’ll seek it out and stuff your face with as much of it as possible. When heated to a liquid, fats are more easily absorbed by items of food, imbuing them with flavor. That’s why fried food tastes awesome, why bakers put butter in their wares, and why cake is absolutely amazing (that and sugar of course).
Still, too much fat can be really bad for your health, and not all fats are created equal, which is why the World Health Organization recommends limiting saturated fat intake to 10% of daily energy intake, less than 7% for high-risk groups, and recommends keeping unsaturated fats below 30% of daily energy intake. As a rule of thumb, animal fats tend to have a higher content of saturated fats, which have the maximum number of hydrogen atoms tied into their chain and only single bonds. Plant-derived fats tend to have a higher content of unsaturated fats — which have at least one double bond and aren’t ‘saturated’ with hydrogen. Saturated fats hold more energy than unsaturated ones.
Proteins
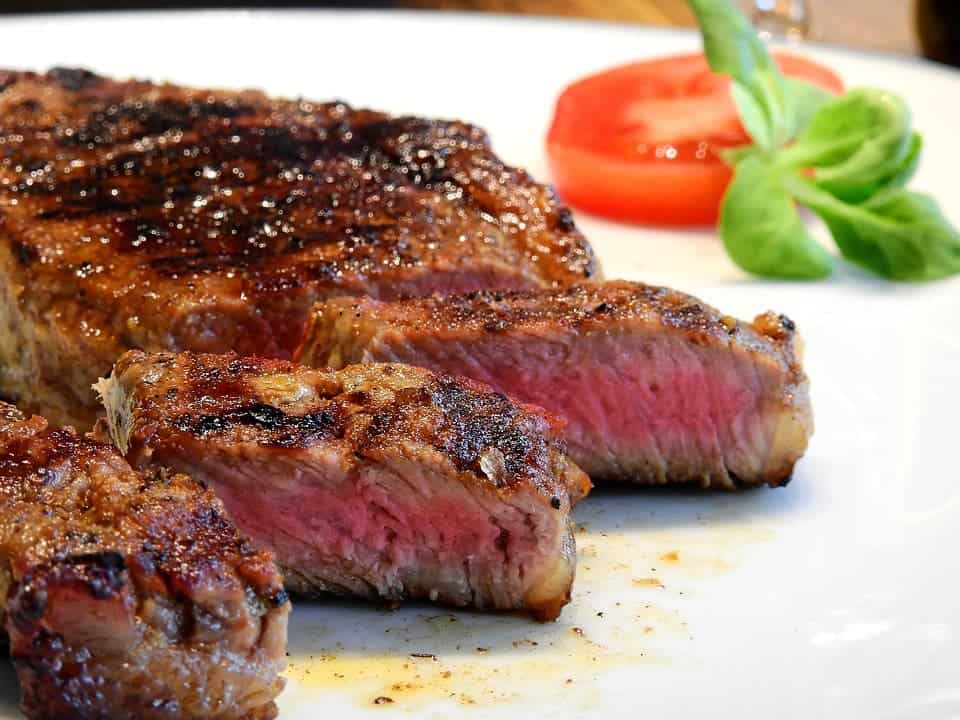
Protein denaturation is, if you’ll pardon the pun, the real meat of chemical reactions in cooking. Proteins are incredibly complicated bits of molecular machinery. They’re what imparts structural resilience to foodstuffs, what makes them chewy.
Proteins have four layers of complexity to them. First is the amino-acid composition itself, which forms the primary structure. Then come the intramolecular bonds between the amino-acids, the secondary structure, the shape the protein is folded in, the tertiary structure, and the overall macromolecule’s 3D shape, the quaternary structure. Their folding patterns are so complex we still have a lot of difficulties replicating them in the lab — despite using supercomputers to crack the problem.
Cooking, however, breaks these layers of complexity down in a process we call denaturation. Essentially, this process reverts proteins to their primary, or at most secondary, structure. This makes the proteins much easier to break down chemically, and less able to hold together mechanically, which is why cooked food is easier to chew or digest than raw food and has more effective calories.
In a way, the process is similar to that of carbs, as proteins are essentially long chains of mostly carbon and hydrogen. Outwardly, however, the effect is kind of the opposite of that in fats. An egg’s white, for example, largely consists of proteins and water. Raw, it’s gooey, runny, slimy, but holds together pretty well. When cooked, the denaturated proteins start interacting with each other and jumbling up, making the white more firm but easier to bite and chew on.
In effect, cooking breaks down the egg white’s proteins and then polymerizes — binds together — the resulting bits. The same process takes place with all proteins in the food you cook, be it an egg or a slab of steak. The Maillard reaction again makes an appearance here. The reactions between proteins and carbohydrates that brown baked goods or toast are the same ones that make meats and other protein-heavy foods brown when cooked.
Taste
What you perceive as ‘taste’ is your brain interpreting the chemical composition of food. Sugars, for example, are sweet, fat gives foods that irresistible, savory flavor, and proteins are generally attributed as the carriers of umami.
But those are the broad strokes. Cooking alters each of these macronutrients, carbs, fats, and proteins both separately and as a whole. On one hand, you have proteins being denatured, on the other you have sugars being transformed, and finally, you have interactions going on between the two in Maillard reactions. Lipids will also seep into the whole thing along with the cocktail of compounds released by sugars and proteins, soaking everything in delicious goodness.
The chemical processes that occur in the pan are extremely complex, and are not completely understood for a simple reason: no two foodstuffs are alike. We have a general idea of the main going-ons while cooking, but it’s simply too large and chaotic an environment to understand fully, — so much so that back in 2015 one of the Ig Nobel prizes was awarded to Colin Raston, a chemistry professor at the Flinders University, Australia, for uncooking an egg.
I’ve tried to keep it lighter on the details than we usually do here on ZME Science precisely because of this huge complexity. However, I hope I’ve helped you get a whole new appreciation of the wonderful chemistry that comes together in a tasty dish. And, if you’re like me, that I’ve something to think about while getting dinner ready.
Now if you’ll excuse me, I’m going to go raid my fridge.