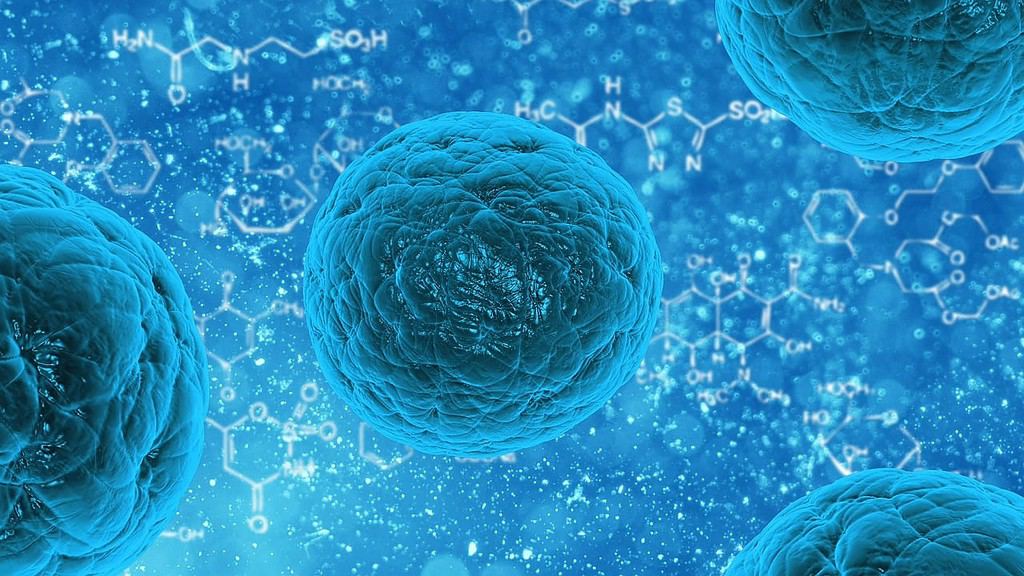
Researchers have made stunning progress in genetic engineering and synthetic biology by modifying a strain of Escherichia coli bacteria to be immune to virtually all viral infections.
While the researchers cannot guarantee that no virus can ever infect this modified strain of E. coli, none of the many different types of viruses they tried was capable of breaching the bacteria.
The technology could reduce the threat of viral contamination when using bacteria as biofactories to produce useful substances like insulin and biofuels. In large vats of bacteria, viral infections can halt production, compromise drug safety, and incur costs of millions of dollars.
By now, you’re probably reading this with mixed feelings of awe and concern. While a virus-free bacterium that escapes into the wild is a scary biological hazard, the scientists took a number of steps to safeguard their genetically modified organism and prevent any unwanted mishaps.
A virus-less strain
The study’s first author, Akos Nyerges, a research fellow in genetics in the lab of George Church in the Blavatnik Institute at Harvard Medical School and the Wyss Institute for Biologically Inspired Engineering, claims that the team has developed the first technology capable of designing an organism that is immune to any known virus.
Nyerges states that although they cannot claim the bacterium is completely virus-resistant, based on extensive laboratory experiments and computational analysis, the team has not found a virus capable of breaking it.
The researchers’ work builds upon previous efforts by genetic engineers to produce virus-resistant bacteria. In 2022, a group from the University of Cambridge believed they had created an E. coli strain that was immune to viruses. However, when Nyerges and colleagues put this claim to the test, they were disappointed to find they could easily infect the modified bacteria.
The team sampled various environments, including chicken sheds, rat nests, sewage, and the Muddy River located near the Harvard Medical School campus. The nasty viruses they isolated were capable of infecting the modified bacteria.
The Cambridge method of blocking viruses from infecting E. coli hinged on cutting down the number of codons in E. coli to 61 from the naturally occurring 64. A codon is a DNA or RNA sequence of three nucleotides (a trinucleotide) that forms a unit of genomic information encoding a particular amino acid or signaling the termination of protein synthesis.
Without the missing codons, a virus shouldn’t be able to hijack the cells of an organism. It’s a pretty brilliant idea, but it wasn’t perfect. Something was missing that was still allowing some viruses to infect the cells.
In addition to deleting codons, the Harvard researchers added a specific type of RNA called transfer RNA, or tRNA for short. This RNA molecule recognizes a specific codon and then adds amino acids to the protein that needs to be built. For instance, the codon TCG tells its matching tRNA that it needs to attach the amino acid serine.
When the Cambridge team deleted TCG, it also removed its corresponding tRNAs. But rather than remove them, the Harvard researchers added trickster tRNA that introduce different amino acids than they should. For instance, when the modified tRNA sees TCG, it adds leucine instead of serine.
“Leucine is about as different from serine as you can get, physically and chemically,” said Nyerges.
When viruses tried to tell the E. coli bacteria to produce viral proteins, the trickster tRNAs added the wrong amino acids, resulting in misfolded, non-functional viral proteins. The virus could no longer replicate and infect more cells.
Although viruses come equipped with their own tRNAs that could turn TCG into serine, the researchers found that the trickster tRNAs they introduced were so effective that they overpowered their viral counterparts.
Now, theoretically, a virus could eventually mutate to overcome the swapped codons and tRNAs. However, Nyerges added that dozens of very specific mutations would be required at the same time to open the back door for the virus. “That’s very, very unlikely for natural evolution,” she says.
Safety first
The technology includes two major safeguards in order to prevent the spillover of these modified organisms into the wild. The first safeguard is meant to offer protection against horizontal gene transfer, which occurs when genetic code snippets and their corresponding traits, such as antibiotic resistance, are transferred from one organism to another. This is distinct from vertical transfer, in which genetic material passes from parent to offspring after they have sex or clone themselves. It’s not uncommon to see bacteria share genes with animals and even plants.
When another organism comes across the modified E. coli and shares trickster tRNAs, the misreading of serine codons will damage or kill the receiving cell, preventing further spread.
“Any modified tRNAs that escape won’t get far because they are toxic to natural organisms,” said Nyerges.
The second fail-safe prevents the bacteria themselves from growing and proliferating outside of a controlled environment. The modified E. coli is 100% dependent on lab-made amino acids that are impossible to find in the wild. If the bacteria were to be taken out of the lab or biofactory and escape, they would die of hunger.
The authors said their technique could have significant implications for biocontainment strategies and the development of GMOs. With viruses no longer a concern, researchers could use modified bacteria to produce valuable biosubstances, such as biofuels or insulin, without the risk of viral contamination. Additionally, the technique could pave the way for the safe deployment of genetically modified crops, reducing the spread of disease and potentially increasing food security.
However, some experts caution that the use of genetically modified organisms, even those with built-in safety measures, still raises ethical and environmental concerns.
Despite these concerns, the HMS team’s breakthrough represents a significant step forward in the field of genetic engineering and synthetic biology. By creating a bacterium that is immune to all known viruses, they have opened up new possibilities for harnessing the power of bacteria to produce useful substances, while minimizing the risk of contamination and gene flow.
We now have a blueprint for making any organism immune to viruses and preventing gene flow in and out of GMOs, paving the way for safer, more effective biocontainment strategies in the future.
The findings appeared in the journal Nature.