A seemingly humble and common device, the air conditioning is in fact the result of crafty engineering. It cleverly uses the laws of physics to move heat from one place to another — out of your house, usually. But as we’ll soon see, there’s no joking around with the laws of physics. It’s a complex topic, so let’s dive in and see how we bend physics to our will for our comfort and safety by squeezing and pumping some strange chemicals around in some pipes.
One thing’s for sure, the inventor of air conditioning didn’t anticipate how far this technology would go.

Keeping things cool isn’t useful just in social situations. In fact, a lot of what we consider to be the modern way of life is only possible because we’ve learned how to make hot things cold. Between 1998 and 2017, for example, more than 166,000 people died due to heatwaves, according to the WHO. Cold conditions seem to be the deadlier overall, as one study reported that between 2011 and 2018 “hypothermia made up 27.0% of all temperature injuries, but 94.0% of all deaths”.
Temperatures can pose a threat through more than just direct exposure. Improper refrigeration of items such as food or medicine can cause them to spoil, leading to financial losses or adverse health effects. Finally, much of our technology needs to be kept within certain temperature ranges to function properly — this includes your laptop, space telescopes, and nuclear reactors.
To understand how we’ve managed to get a grip on temperature control, let’s first start with the basics.
What is ‘temperature’?
Temperature is how we measure how much thermal energy something has. It’s closely related to, but not the same thing as, the concept of heat.
Now, if you had a powerful enough microscope and looked at an object that’s heating up, you would see its molecules or atoms vibrate ever more intensely. This motion, ultimately, is thermal energy. Just like a wind chime in motion produces louder sounds the more its parts collide, an object’s particles generate more thermal energy the more they move. This type of particle activity is known as Brownian motion.
What we perceive as ‘heat’ is a transfer of this energy. Concepts of ‘hot’ and ‘cold’ are only applicable in relation to something — for example, a cup of boiling tea is hotter than a cube of ice but much colder than the sun. In order for you to perceive an object as hot or cold there needs to be some way for that energy to transfer between the object and your body. If it has less energy it will draw some away from your body, and your brain tells you it’s cold. If it gives you energy, you perceive it as hot.
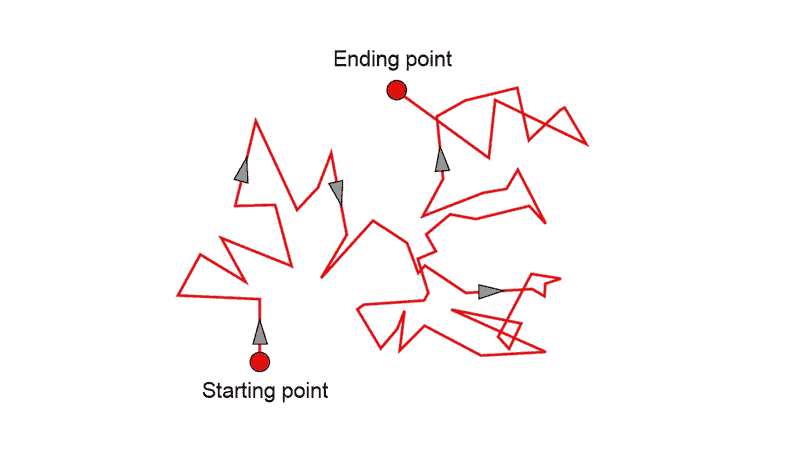
In general, all bodies exchange heat with those around them (in a physical sense, even the atmosphere, the planet, or the universe are bodies) as long as they are in thermal contact. This ultimately leads to heat being more or less equally distributed in a system — hence why we have the idiom of something being “room temperature”. It’s everything in the room, from the table to the air itself, sharing the same heat energy so they will all feel about the same temperature to us.
Broadly speaking, we measure thermal energy using two units of measurement: the British thermal unit (‘Btu’ or ‘B. Th. U.’), or the French thermal unit (the ‘calorie’). They both largely function the same way, describing how much energy is needed to heat up a certain quantity of water by a certain temperature. However, they differ in how they measure these. One Btu represents the thermal energy needed to raise the temperature of one pound of pure water by one degree F. The calorie uses that most unholy of constructs instead — the metric system, — and describes how much energy you need to heat up one kilogram of pure water by one degree C.
Okay, so quick recap. Temperature is a way to measure the internal energy of a body, and that energy manifests as movements on the molecular and atomic levels. What we feel as hot and cold is a flow of this energy from one body to another. In general, all bodies that come into contact try to equalize their internal heat levels.
Doing a hot take
Given that thermal energy has a corresponding, physical representation in the movement of particles, it stands to reason that if you can make them stop, you can cool down an object. The fundamental problem with this, however, is that heat is the residual form of energy in our universe. Every other type of energy eventually will degrade into thermal energy through physical work, but we can’t run the process in reverse and turn thermal energy into another type of energy directly.
The motions associated with thermal energies are chaotic, and carry extremely low levels of energy individually — making it impossible to ‘harvest’ it to do physical work (due to entropy). This is why mechanisms like steam engines use heat (a ‘flow of thermal energy’) instead.
Now, from what we’ve seen so far, it seems that the way to cool down a glass of water is to cool down a room, and the best way to do that is to cool down the planet. Needless to say, we’re doing the exact opposite today and yet still get our chilled beverages. We have two main ways of doing this: ventilation and refrigeration.
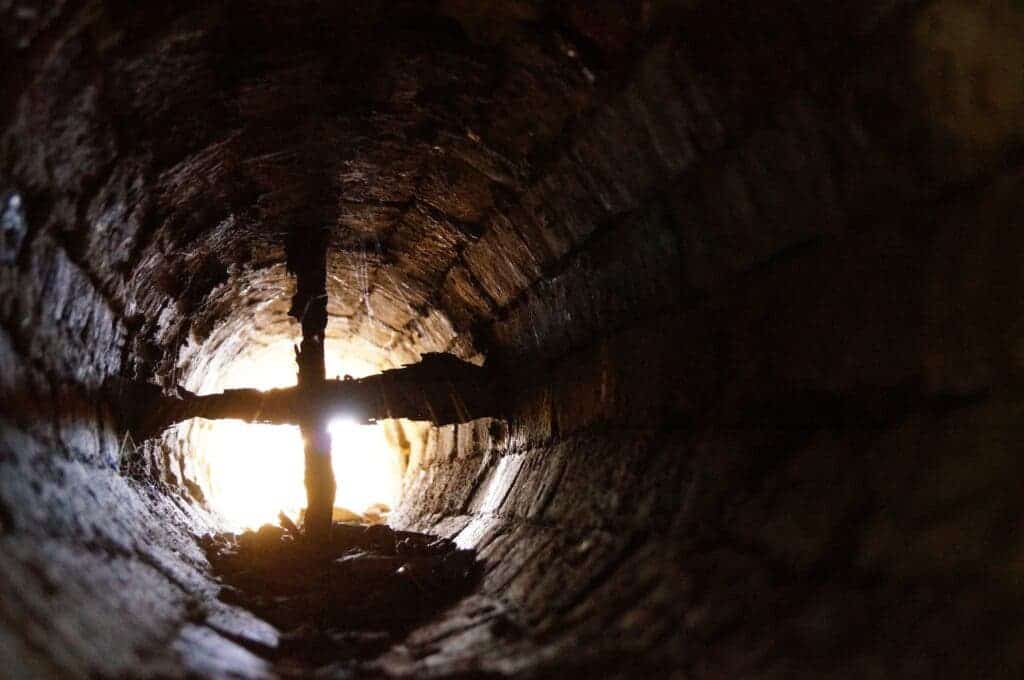
Ventilation is the simpler approach, and we’re not the only ones to do it. In essence, ventilation relies on heat imbalances between two physical bodies to move masses of a medium (usually air or water) around. Because thermal energy is represented by molecular movements, having a current of air go over an object will lower its temperature as these vibrations are transferred to air molecules and carried away. Ventilation is how your computer keeps cool, and it is a part of how air conditioners work, as well.
The main limitation of this process is that it stops working when the object reaches the same temperature as its environment. At that point, the transfer of heat can stop altogether, or change directions from the environment to the body itself, heating it up.
This being said, a flow of air can provide a cooling effect even when the medium becomes quite hot. That’s why the breeze is soothing even on a scorching hot day, and how termite nests keep cool even in the hottest conditions.
Refrigeration is more technically challenging, but it can be used to lower an object’s temperature below that of the ambient environment. The heat removed from this object must be dumped into an area with a higher temperature, meaning energy must be expended in the process (as it creates a physical imbalance). It is this process that allows your air conditioner to hold a certain temperature, your freezer to freeze, and so on. The secret behind this process lies in manipulating another physical parameter: volume.
Squeeze for hot, relax to cool

First, you need to know that refrigeration systems need a special medium, known as the refrigerating fluid or agent, to work. This agent will physically carry heat from one part of the system to another. The requirements for a good refrigerant are a low boiling point, a relatively low density in liquid form but a high one as a gas, and that it has a high heat of vaporization (it can absorb a lot of heat before turning into a gas).
The magic happening inside a refrigeration unit hinges on changing the pressure of this fluid along the refrigeration system. At one end of the system, a component known as the compressor squeezes the agent hard, lowering its volume. This step causes it to heat up rapidly (because it holds the same amount of thermal energy but in a smaller place — more collisions happen). Although it’s becoming compressed, the fact that it’s heating up keeps this fluid in a gas state. It’s important that the process does not result in the refrigerant becoming a liquid, since liquids can’t be compressed, and this would damage the system.
As it leaves the compressor, the refrigerant is a hot vapor, at roughly 120° to 140°F (48° to 60°C).
The high-pressure fluid is then allowed to exit the compressor and naturally flows to areas of the system where pressure is lower. The next component it flows into is called the condenser or outdoor coil. Here it is allowed to cool down by passing heat off to the environment. Because the agent coming into the coil is so hot it will still naturally pass off its thermal energy even when it’s hot outside. This is why the back of your fridge is always so hot, or why air conditioning units blow a current of hot air.
The fluid is kept pressurized in this component, but it’s still too hot to turn liquid. As it exits the coil, however, it is fed through an expansion valve. This component allows it to turn into a low-pressure liquid through a process known as flashing. If you’ve ever used a can of compressed air or a fire extinguisher, you’ll know that fluids become significantly colder when the pressure drops / they increase in volume. This is the step that actually cools the fluid down enough to be useful for refrigeration.
Now a liquid, it is fed through the evaporator. This takes the shape of pipes inside the fridge, for example. Being very cold at this point, it absorbs heat, essentially draining thermal energy from the surrounding environment and starts boiling. In an air conditioner, a fan blows air over the pipes or radiator containing this liquid to pump cold air into the room.
The fluid, now back in a gas form at roughly room temperature, is pumped back into the compressor and the cycle repeats.
Reverse perspiration
When we sweat, water on our skin evaporates — it increases in volume — which makes it drain thermal energy from our bodies. This cools us down and makes our environment more humid.
The evaporator works in reverse. Water condenses on these cold surfaces, meaning it cedes its own thermal energy to become a liquid. This heats the refrigerant up and makes the environment very dry. Air conditioning systems are thus also able to dehumidify air in a room or to control humidity levels.
Refrigeration works because all the individual steps — compression, condensation, and evaporation — are forced to happen in different places, which shifts thermal energy around. So whenever you’re using your air conditioner at home, know that it’s not really ‘absorbing’ heat, it’s just taking it from inside and dumping it outside. Thus, fridges, freezers, and air conditioners are a great example of the First Law of Thermodynamics, that energy cannot be created or destroyed.
But it can, with some tricky engineering, be moved somewhere else to make us all more comfortable and safe.