Curious enough, one hundred years after renowned physicist Max von Lauefirst used X-ray diffraction to unravel the intricate atomic architecture of molecules, a team of international scientists have analyzed tiny protein crystals at an unprecedent scale of resolution, premiering in the process the world’s first hard X-ray free-electron laser. Called the Linac Coherent Light Source at Stanford, the X-ray laser was made possible after a 300 million dollars investment from behalf of the US Department of Energy.
Most of our current knowledge regarding the 3D spatial architecture of molecules has come as a result of X-ray crystallography, a field of science which has seen much progress in the past few decades, making possible equally amounts of achievements in molecular analysis. Crystalography basically studies the spatial arrangements of atoms in solids. Modern crystallography relies on the amplification of the scattering signal of the molecules by their arrangement into relatively large crystals, often on the order of some tenths of a millimeter.
Large crystals, extremelly helpful for accurate analysis, are very difficult to obtain, however, especially in the case of bio-molecules due to instability and low abundance. This is where free-electron lasers come in, revealing structural information from crystals otherwise unobtainable through conventioanl methods, since radiation irremedially damages them before anything useful can be drawn.
The innovative X-ray free-electron lasers are new X-ray sources of extreme potency, capable of releasing high intensity ultrashort flashes of light. The intensity of such an X-ray pulse is more than a billion times higher than that provided by the most brilliant state-of-the-art X-ray sources, with a thousand-fold shorter pulse length, on the order of a few millionths of a billionth of a second, or femtoseconds. These properties provide scientists with novel tools to explore the nano-world, including the structure of biological materials.
This extremely high frequency of firing light flashes allows the X-ray free-electron laser to record information from the sample before damage irremediably occurs. The crystals samples are destroyed in the process, much like by conventional means, but it’s so fast it gets what it needs from the crystal before interferring damage gets a change to disrupt analysis.
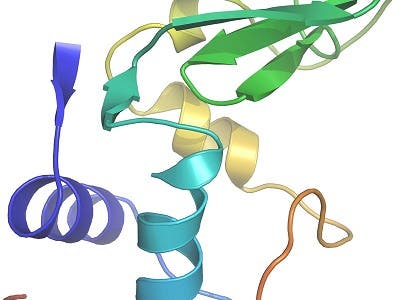
To benchmark the method, the researchers investigated the structure of an exhaustively studied molecule, the small protein lysozyme, the first enzyme ever to have its structure revealed. Ten thousand snapshot exposures from crystals that measured only a thousandth of a millimeter, showed that the data compared well with those collected using conventional approaches and hundred-fold larger lysozyme crystals.
“This proof-of-principle experiment shows that the X-ray free-electron laser indeed lives up to its promise as an important new tool for structural biology on large macromolecular assemblies and membrane proteins. It really opens up a completely new terrain in structural biology”, Ilme Schlichting, leading the Max-Planck team, says.
The reserach was spearheaded by scientists at the Max Planck Institute for Medical Research in Heidelberg and the Max Planck Advanced Study Group in Hamburg. The findings were reported in a recent edition of the journal Science – you can read more about it in the magazine.