The observable universe contains an estimated 1×1024 stars. They come in very different sizes, and their masses and brightness can vary dramatically. Even so, researchers have developed a system that managed to look at them all together.
The most common classification system is called the Morgan–Keenan (MK), which classifies stars based on temperature and luminosity. The MK system essentially merges two different systems:
- the Harvard classification, where stars are classified on their surface temperature using a single letter of the alphabet: O (the coldest), B, A, F, G, and M (the hottest). These are the so-called main-sequence stars, but as we’ll see, there are also more extreme types of stars (like giant stars, supergiant stars, and white dwarfs). These broad types are also split, so you can have G1 stars, G2 stars, and so on.
- the Yerkes spectral classification (sometimes used synonymously with the MK system), which is luminosity, where stars are named using Roman numerals: 0, I (Ia, Iab, Ib), II, III, IV, V, VI, and VII.
In the MK system, stars are defined with a letter from the Harvard classification and a Roman numeral from the Yerkes one (the Sun is a G2-V star).
Over the years, astronomers have created different catalogs where stars are classified in somewhat different ways, and it’s not always a straightforward process, nor is the classification set in stone — it has changed over the years, as our understanding of stars has improved. So if anything, the stellar classification discussed is a general indication more than anything else.
Now, let’s look at things in a bit more detail and see why this matters.

A star is born
The universe is big, dark, and cold — the vast majority of it, at least.
But if you were to wander this universe, you’re bound to come across something that’s hot and bright. Stars, as these objects are called, are giant thermonuclear reactors. They’re responsible for heating up some planets to a livable temperature, they produce the vast majority of the chemical elements we know of, and they’re basically the reason why the universe is not a completely frozen and barren place.
A star’s life begins when a gaseous nebula of material starts to collapse on its own gravity.
Just like planets, all stars are round, because of this gravitational collapse. When the stellar core gathers sufficient mass, it starts to develop a significant gravitational attraction, which packs the particles even more tightly, increasing the density. Then, once a crucial point is reached and the stellar core becomes sufficiently dense, hydrogen starts to be converted into helium through nuclear fusion, releasing energy in the process — and thus, a star is born.
This hydrogen-helium fusion is the fuel of the star, and the heat it produces also creates a pressure that prevents stars from collapsing on themselves. Depending on how massive they get, stars will also end up on a different course.
In general, larger stars have shorter lives, although we’re still usually talking about billions of years. However, their lifecycle greatly depends on their mass.
For instance, a star with a mass greater than 0.4 times the Sun will expand and turn into a red giant. Then, as it ejects much of its mass violently across the galaxy, the star would become a white dwarf. Based on its mass, the star might also become a brown dwarf, a neutron star, or, if it is sufficiently massive, a black hole. So already, we’re seeing that different types of stars can turn into different things after they use up their fuel.
- Average stars become white dwarfs. They eject their outer layers but still maintain a hot core comparable in size to the Earth. Counterintuitively, the smaller the white dwarf, the larger its mass — this happens because it’s the pressure from fast-moving electrons that keeps these stars from collapsing, so the more massive the star, the more it is able to overcome this pressure, and thus, massive white dwarfs tend to become smaller.
- White dwarfs can become active stars once again. If a white dwarf is close enough to a companion star, it can start sucking material from the second star, and if it acquires enough, it can produce a burst of nuclear fusion, causing it to brighten and turn into a nova. This typically lasts a few days, after which the process starts again. If enough material is gathered in one go, the white dwarf can erupt into a supernova.
- Larger stars become supernovae. A supernova is not only a big nova. In a nova, the star’s surface explodes but in a supernova, the core also collapses and explodes. The supernova-generating process is cataclysmic — in mere seconds, the core shrinks from thousands of miles to only a few dozen, and the temperature can spike by over 100 billion degrees. A supernova eruption can outshine an entire galaxy. Every year, we discover 20-40 supernovae in other galaxies.
- Supernovae can become neutron stars. After this ungodly process, the core collapses. If the collapsing stellar core has a mass of 1.4-3 solar masses, what’s left behind is a neutron star. Neutron stars are the smallest and densest stars, if we exclude black holes or hypotheticals such as white holes. Neutron stars have a radius on the order of 10 kilometers, and the ones we’ve observed so far seem very hot, although they don’t actually produce heat anymore and slowly cool down over time.

- Large supernovae turn into black holes. If the stellar core remaining after the supernova explosion is larger than three solar masses, it will collapse into a black hole. Black holes are the most massive objects we know of in the universe, so massive that even light cannot escape their grasp. Black holes are so exceptional that they start to break our current understanding of physics. Black holes are thought to be extremely cold on the inside, but incredibly hot just outside.
- Stellar debris often forms new stars. The dust and debris ejected by novae and supernovae are excellent seeds for new stars, recycling the material and potentially forming new generations of stars.
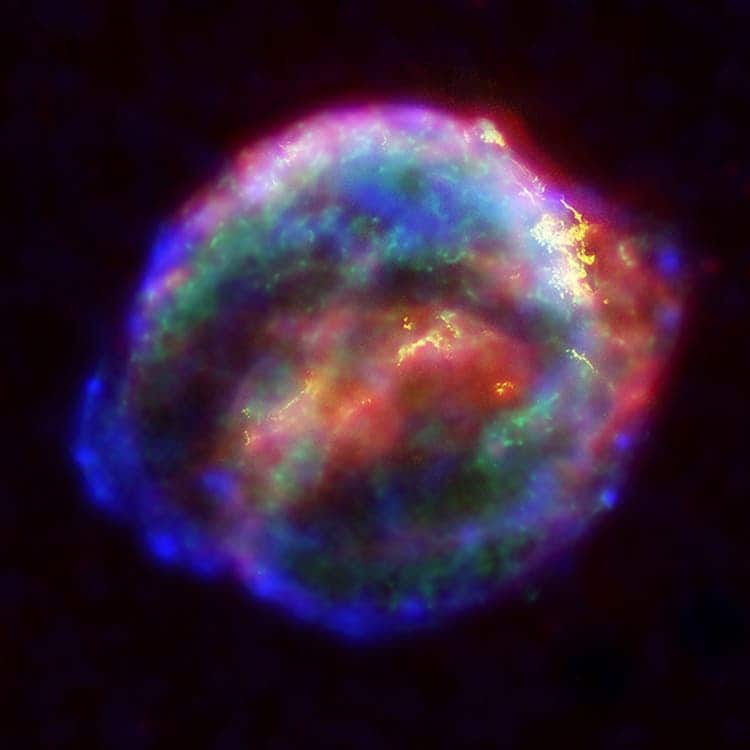
Understanding stars
We’ve already seen some types of stars but before we dig into the classification per se, let’s take a brief look at what we know and don’t know about stars.
Outside of the sun, the closest star to Earth is one of the three stars in the Alpha Centauri system, some 4.3 light-years from Earth. That’s very, very far. To get an idea of how far it is, the distance from Earth to Mars is around 0.00001 light-years away and, with our best available space ships, it would still take around 7 months for the trip. To get to Alpha Centauri at that speed, it would take over 100,000 years. So while mankind has looked at the stars forever, we’ve only recently started to look at them closely.
Of course, we’re not physically close to the stars, but with the aid of telescopes, we can get a closer look at them. Ground-based telescopes enable scientists to study stars in different wavelengths of light, including the visible spectrum, radio waves, and even infrared light.
It’s not just observation — researchers also conduct experiments in the lab to infer the properties of stars and investigate the processes that fuel stars. Ultimately, computer modeling has also helped improve our understanding of stars.

With computer models, we can approximate different properties of stars (such as density, pressure, velocity, and composition) and see how they influence observations.
Enough chit-chat. Let’s look at some stars.
Main sequence stars — and beyond
In astronomy, the ‘main sequence‘ is a term used to denote stars that fit in the common group of stars on the color versus luminosity charts. These plots, also called Hertzsprung–Russell diagrams after their co-developers, are the common classification tool for stars.
The Hertzsprung–Russell diagram is essentially a square where stars are plotted with color on the horizontal and luminosity on the vertical. Color is dependent on temperature, and brightness depends on size so you can also see it as a temperature-size chart.
The main sequence of stars is exactly what the name says: the main sequence in which these stars are grouped, from top left (bright and blue/hotter) to bottom right (less bright and less hot).
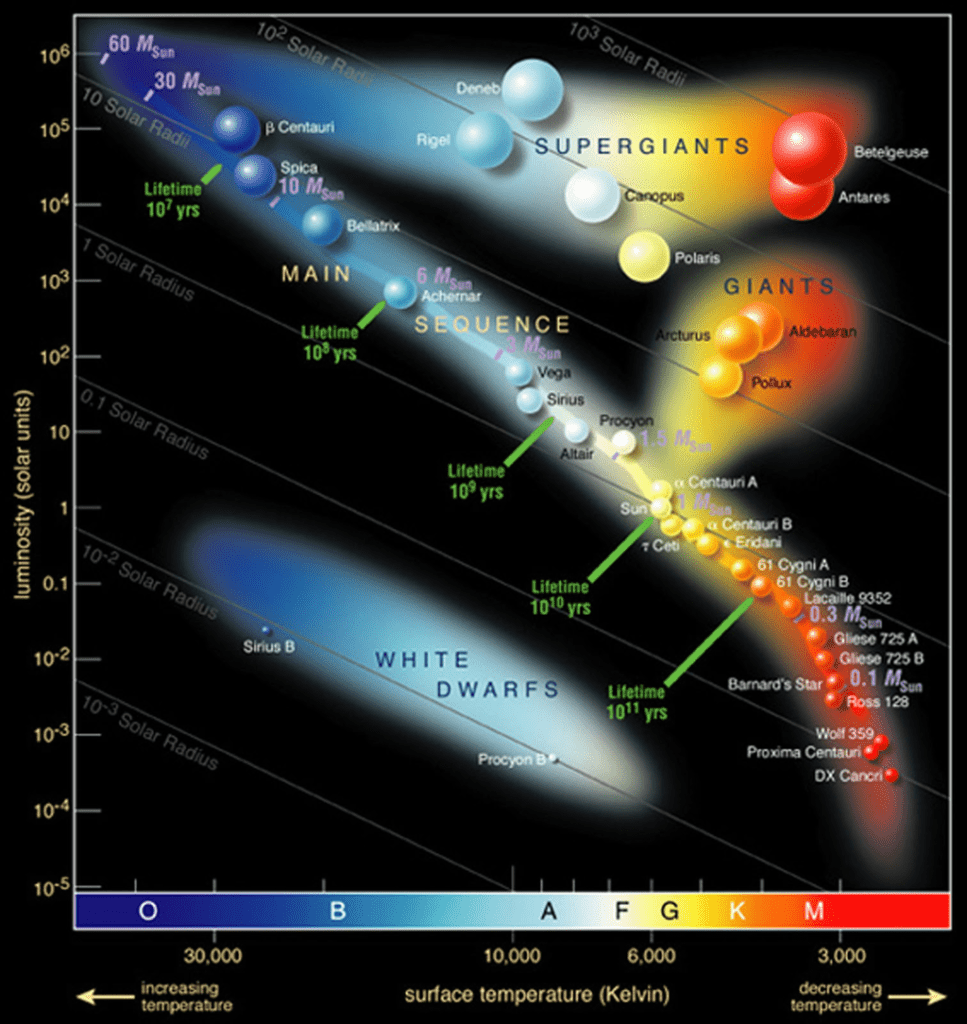
It’s hard to say why stars are grouped along this chart, but about 90% of all the known stars in the universe (including the sun) are main-sequence stars.
That doesn’t mean that main sequence stars are monotonous — quite the opposite. There can be huge variations, ranging from a tenth of the mass of the sun to up to 200 times as massive.
There are also other clusters of stars — to the left, we see the less bright white dwarfs, which we’ve discussed already, and above the main sequence, we have the giants and supergiants. Here’s what a real diagram of stars looks like, from 22,000 stars plotted from the Hipparcos Catalogue and 1,000 from the Gliese Catalogue of nearby stars, with the most prominent being the main-sequence diagonal.
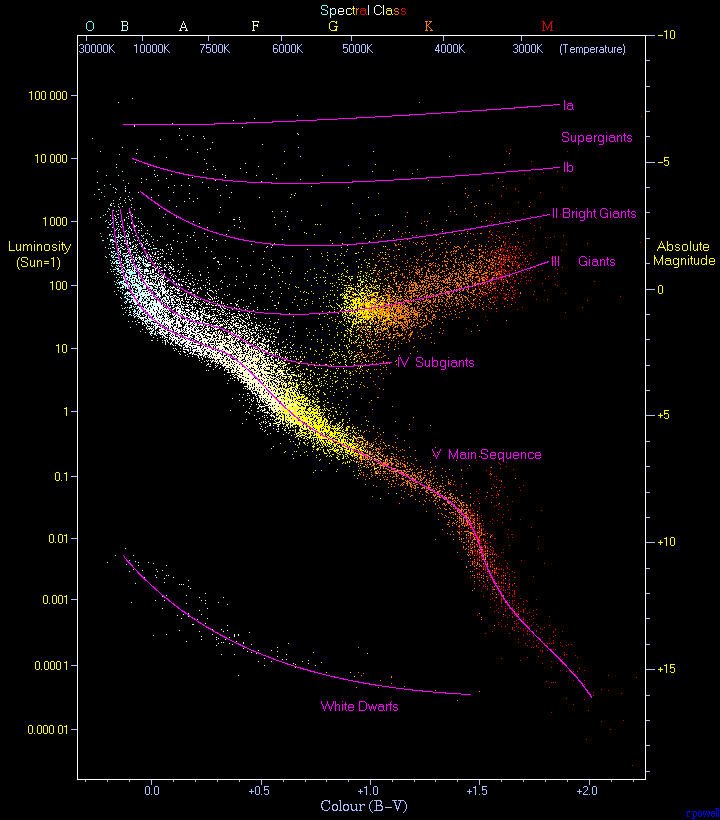
So the first stellar classification is into main-sequence stars and non-main-sequence stars. Main sequence are stars are the norm, other types of stars can exist scattered around the diagram (though they also tend to group into clusters).
Types of main sequence stars
As we’ve already mentioned, main sequence stars are also extremely varied, so there is also a separate classification for them.
The traditional classification here is called the Harvard classification, a one-dimensional classification where stars are grouped by their temperature, with a letter representing each category. However, this classification does not distinguish between stars with the same temperature but different luminosities. So a new classification system was devised — the Morgan–Keenan (MK) classification, which also includes the luminosity.

The modern classification system for them is called the Morgan–Keenan (MK) classification and also includes luminosity.
Each star is assigned a spectral class from the older Harvard spectral classification, as well as a luminosity class using Roman numerals as explained below, forming the star’s classification. So you’d have, for instance, B0 stars, BI, stars, BII, and so on.
Ia-O | extremely luminous supergiants |
Ia | luminous supergiants |
Ib | less luminous supergiants |
II | bright giants |
III | normal giants |
IV | subgiants |
V | main sequence dwarf stars |
VI, or sd | subdwarfs |
D | white dwarfs |
So M stars are the smallest, O stars are the biggest, and the luminosity ranges from I (brightest) to VI (less bright) and D (white dwarfs). As mentioned, the Sun is a type G2V star. Alpha Centauri A is also a G2V star, while Proxima Centauri is a type M5.5-V star.
The classification can also be expanded to stars outside of the main sequence. A plot of the stars would look like this:

Why we classify stars
Well, for starters, researchers love to classify all things — that’s how we better understand trends and patterns. That’s how we know, for instance, that M type stars are by far the most abundant in the known universe. The Sun belongs to a relatively rare group of stars.

Astronomers also calculate the habitable zone around different types of stars — the distance at which a planet can exist around the star so that it hosts liquid water, and therefore, could potentially host life.
NASA’s Kepler mission is searching for habitable planets around nearby main-sequence stars less massive than type A stars but more massive than type M — so mostly around K, G, and F types of stars.
Ultimately, we class stars because we want to better understand and define them. We’re still only scratching the surface of a very vast universe of knowledge, and it helps to group things in an orderly, systematic fashion.
More exotic stars
It’s not the only stellar classification, and it’s not entirely fixed and set in stone. There are other classifications and talk about changing classifications is not unheard of in the astronomical world, as our understanding progresses and improves.
There are also more exotic types of stars. For instance, recent research has suggested that Class L (small, dark, reddish) stars and Class T (methane dwarf) stars could be more common than all the other classes combined, but they are more difficult to discover.
There is yet another class — Class Y of brown dwarf stars, cooler than those of spectral class T and with different spectral signatures. So far, less than two dozen of these stars have been confirmed. Class C of carbon stars is also discussed, and Class D stars is typically used to denote any stars that are not currently undergoing fusion. It’s estimated that there are 300 billion stars in our galaxy alone, and we have very little idea how many galaxies there are in the universe, but estimates range in the trillions. I’ll let you do the math.
As our detection capacity and image processing power advance, we are likely to keep discovering new stars that improve and challenge our current understanding. There is much, much more to discover.
