We are now learning new details about how the HIV infects cells thanks to a massive simulation of the viral capsid. The capsid is a protein cage that protects the viral genome and delivers it to infected cells.

To get an idea of the sheer complexity of this project, the team led by Juan Perilla and Klaus Schulten, both at the University of Illinois, performed a 64-million-atom simulation of how the virus senses its environment and completes the infective cycle. Even so, we’re still scratching the surface. It took them two years to simulate just 1.2 microseconds in the life of the HIV capsid.
Viruses come in all shapes and sizes. Some are simple protein shells filled with RNA or DNA while others are surrounded by a membrane which rivals cells in complexity. HIV is one of these really complex viruses which is surrounded by a membrane and filled with viral and cellular molecules. HIV’s viral genome is comprised of two strands of RNA packaged inside a cone-shaped capsid.
The HIV capsid is made from a single type of protein called the capsid protein, also known as CA or p24. The capsid folds to form two domains connected by a flexible linker given the protein enormous flexibility enabling it to fold in numerous possible configurations. Hundreds of identical proteins link up to form the HIV capsid. The larger domain associates with other copies of the protein to form rings of six, and slightly less often, rings of five. The smaller domain then links these rings together to form the larger structure.
It’s this inherent complexity and flexibility of the HIV virus that has always proven challenging to researchers. Building on a method called ‘computational microscopy’ pioneered by professor Schulten, the team was able to not only peer into the structure of the HIV capsid but also learned how it responds to its biological environment. Sadly, Schulten passed away in October 2016 before this paper was published in Nature Communications.
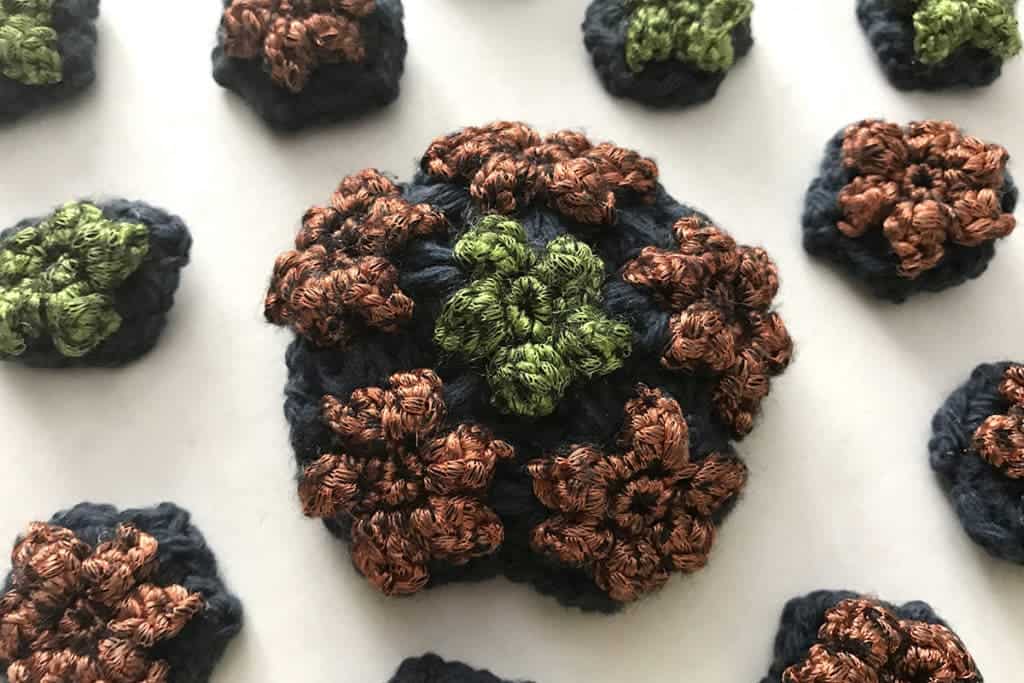
The simulation was run on the Titan supercomputer operated by the Department of Energy, with help from a second supercomputer called Blue Water housed at the University of Illinois’s National Center for Supercomputing Applications.
According to the new study, there are likely several properties that enhance the capsid’s ability to better navigate its environment and, eventually, find its way into a cell’s nucleus. For instance, different parts of the capsid oscillate at different frequencies, which likely serves to communicate information from one part of the capsid to another. Each capsid ring has a tiny pore at its center and the study showed ions flow into and out of these pores. Negative ions accumulate on the positively charged surface of the capsid, while positive ions adhere to the outside, which carries a negative charge. This may be an exciting valuable insight to scientists toiling to find a way to destroy the virus.
“If you can break this electrostatic balance that the capsid is trying to keep together, you may be able to force it to burst prematurely,” Perilla said.
That’s not all. The positively charged interior of the pores could facilitate the influx of DNA building blocks called nucleotides, which carry a negative charge and are small enough to pass through the tiny pores. The nucleotides are essential so the virus can use them to convert its own RNA into DNA. What’s more, stress seems to propagate through the capsid in patterns and some regions are more susceptible to bursting. These are all quite a few new vulnerabilities that we’ve learned and could use them to our advantage in the fight against HIV.