An international research effort to construct the first fully synthetic yeast is well under way. The scientists have fully designed the fungus’ genome and have already built five of its final sixteen chromosomes — planning to have the rest completed by the end of the year.
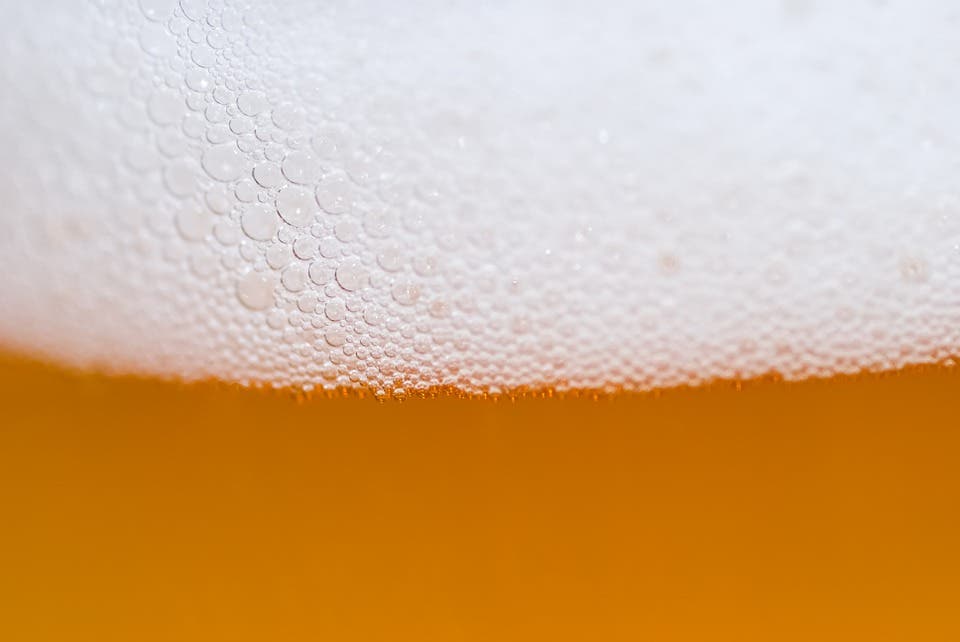
Yeast has to be humanity’s favorite fungus. Sure, other shrooms taste better in a saute or make for a much more entertaining way to spend some free time, but yeast has been by our side since times immemorial. Whenever we’ve needed something fermented, yeast had our back. Without it, there would be no alcohol, no bread, no fish sauce!
Since modern industries need to ferment more stuff much faster and into a more varied range of end products than ever before (think biofuels, insulin, antibiotics, THC), scientists have spent the last two decades sequencing yeast genome to produce different strains useful for all these products. That still leaves us limited by much of the yeast’s genome, however, which nature sadly didn’t design for industrial applications — but not for long.
Led by NYU Langone geneticist Jef Boeke, PhD, and a team of more than 200 authors, the Synthetic Yeast Project (Sc2.0) has designed a full genome for a functioning synthetic version of Baker’s yeast (S. cerevisiae). The latest issue of seven papers coming from the group shows that they’ve successfully constructed almost one third of this genome — 5 out of 16 chromosomes. They plan to have the rest ready by the end of the year. The new round of papers consists of an overview paper and five individual ones describing the first assembly of synthetic yeast chromosomes synII, synV, synVI, synX, and synXII. A seventh paper provides a first look at the 3D structures of synthetic chromosomes in the cell nucleus.
“This work sets the stage for completion of designer, synthetic genomes to address unmet needs in medicine and industry,” says Boeke, director of NYU Langone’s Institute for Systems Genetics.
“Beyond any one application, the papers confirm that newly created systems and software can answer basic questions about the nature of genetic machinery by reprogramming chromosomes in living cells.”
Learning the A’s and C’s
Apart from the immediate utility of having a tailorable yeast strain to apply in industry, Baker’s yeast was selected because of it’s relative simplicity and similarity to human cells. Sc2.0’s researchers are akin to a group of genetic programmers — they add or remove parts of DNA from chromosomes to dictate new function or prevent diseases or weakness to various factors. It makes sense to start with a simple ‘program’ until you learn the basics, which you can then apply to more complex systems.
Three years ago, Sc2.0 successfully assembled the first synthetic chromosome (chromosome 3 or synIII) out of 272,871 base pairs — the blocks which make up DNA. This process starts with the researchers screening libraries of yeast strains to find which genes are most likely to have useful features. Then, they planning thousands of permutations in the genome in a process somewhat similar to very rapid evolution. Some of these changes introduce the new genes to make the yeast exhibit desired features, others remove bits of DNA which were shown not to have a function in past trials.

Image credits Doc. RNDr. Josef Reischig, CSc.
After the computer models are finished, the team starts assembling the edited DNA sequence bit by bit until they have the whole thing. The completed sequences are then introduced into yeast cells, which handle synthesizing and finish building the chromosomes — the latest round of papers describes a major innovation in this last step.
Until now, the researchers had to finish building once piece of a chromosome before work could begin on the latter, severely limiting their speed. These sequential requirements bottle-necked the process and increased cost, Boeke said. So the team made efforts to “parallelize” chromosome assembly, with different labs around the world synthesizing different bits in strains which were then mated. The resulting yeast strains would in some instances have even more than one fully synthetic chromosome. A paper led by Leslie Mitchell, PhD, a post-doctoral fellow from Boeke’s lab at NYU Langone, described the construction of a strain containing three synthetic chromosomes.
“Steps can be accomplished at the same time in many locales and then assembled at the end, like networking laptops to create a global super computer,” says Mitchell.
Another paper describes how a team at Tsinghua University used the same parallelized method to synthesize chromosome synXII, which formed a molecule with more than a million base pairs (one megabase) in length when fully assembled — the longest synthetic chromosome ever made by humans. It’s still only 1/3,000 the length of a human chromosome, but it’s closer than we’ve ever come before.
The researchers also found that they can edit some dramatic changes into the yeast genome without killing the cells. They survived even when the team moved whole sections of DNA from one chromosome to another, DNA swaps between yeast species, often with very little effects on the cells.
There’s a huge potential to synthetic yeast. Scientists could tailor their genome to produce anything we need from drugs, to food, new materials, almost anything — just from sugar and raw materials. It could fundamentally change how we think about a lot of industries, potentially churning the same products as factories and labs from a humble barrel.
But the work performed under the Sc2.0 project also revolutionizes how we know about genome building and synthetic life. Yeast is simple, but the end goal is to one day move on to tailor-made plants, maybe even to perfect the human genome. But we’re still a long way from that. Right now, the team will focus on getting their yeast’s final A’s, T’s, G’s, and C’s in place.