Researchers at MIT found that individual cells in a bacterial colony will grow in a manner that is beneficial to the whole culture, even if this comes at a personal expense for the cell. With this in mind, it appears seemingly complex colony structures can be explained by an essentially simple behaviour. The findings could aid researchers understand how bad bacteria growth can be halted or destroyed, and aid in drug testing.
“Once cells make that decision to live as a communal set of organisms, what other things do they have to start doing to make living as good as possible?” asks Chris Kempes, who did much of the work as a graduate student in MIT’s Department of Earth, Atmospheric and Planetary Sciences. “Once you enter into that intimate cooperation with neighboring cells to benefit the group, it starts to hint at becoming complex, multicellular life.”
For the greater good
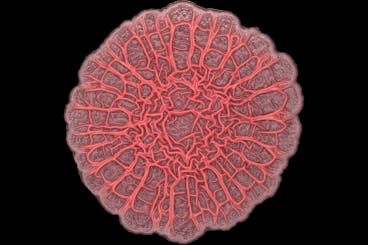
Kempes and colleagues studied a particular strain of bacteria called Pseudomonas aeruginosa, typically found in both soil and water, and known to cause potentially lethal lung infections in humans (cystic fibrosis). The authors set-out to culture this bacteria in the lab on solidified agar. Initially, the cells clumped together to form a smooth disk that continued to grow outward across the growth medium. After a while the cells began to grow upward too, forming wrinkles in a radial pattern as each wrinkle expanded in both height and width. From a certain limit point, however, the culture stopped growing in width and exclusively continued to grow in height.
The researchers presumed oxygen played a major role in this behaviour, so they decided to test this idea by performing a mathematical simulation. The model accurately rendered the distribution of oxygen within a community, compared with the group’s experimental results. Then, by varying the width of wrinkles in the model , the researchers found that for a given amount of oxygen, there exists an optimal width to which wrinkles will grow in order to maximize a colony’s survival; any wider or narrower, and the entire community grows less quickly.
Back in the lab, the researchers grew more colonies under variable oxygen levels: 15%, 21% (Earth atmosphere) and 40%. Indeed, the colony adapted accordingly to oxygen levels, growing narrower wrinkles when there was less oxygen, and wider when more oxygen.
“It turns out the morphology of the colony can be clearly related to something that might do with the fitness of the colony,” Mick Follows says. “It’s very beautiful to see that in a simple way.”
Lars Dietrich adds, “In principle this could apply for most bacteria and even organisms from other domains of life, including microbial eukaryotes.”
Growing strong
Moreover, taking their experiments further, the MIT researchers also found there’s a genetic component that influences the bacterial colony’s growth structure and rate. In one experiment, they grew a culture of P. aeruginosa that lacked phenazines— chemicals that mimic the effect of oxygen, effectively helping a cell to breathe. Apparently, these mutant bacteria grew narrower than the wild kind suggesting that more oxygen-like resources encourage such growth. A second mathematical model simulating the wild strain, developed by Kempes, showed similar results.
The present research provides valuable information on the spatial growth pattern of bacteria and may prove to be useful in following studies looking to battle certain bacterial diseases. Also, the model be useful in determining how a colony grows in the presence of certain chemicals or drugs, such as those that target cystic fibrosis.
“There is a growing literature on how bacterial populations can be used as model systems to understand other populations, such as cancer [cells],” says Joao Xavier, a computational biologist at Memorial Sloan-Kettering Cancer Center,, who was not involved in the research. “This paper makes an important contribution because it shows that simple metabolic processes can explain spatial structure that looks quite complex.”
“You could imagine searching for a chemical that disrupts a cell’s ability to figure out the optimal geometry, so they would die,” says Kempes, adding that while it may be difficult to limit a colony’s oxygen intake without also starving the lung, the group’s model and experiments “open up a playground for starting to think about testing different drugs.”