
Ever since former NASA engineer Kirk Sorensen revived the forgotten molten salt reactor (MSR) technology in the 2000s, interest in MSR technology has been growing quickly.
Since 2011, four separate companies in North America have announced plans for MSRs: Flibe Energy (started by Sorenson himself), Transatomic Power (started by two recent MIT graduates), Terrestrial Energy (based in Canada, which recently partnered with the Department of Energy’s Oak Ridge National Laboratory), and Martingale, Inc., which recently made public its design for its ThorCon MSR.
In addition, there is now renewed interest in MSRs in Japan, Russia, France and China, with China also announcing that MSR technology is one of its “five innovation centers that will unite the country’s leading talents for research in advanced science and technology fields, according to the Chinese Academy of Sciences.”
Why this sudden interest in nuclear technology that dates back to the 1950s? The answer lies in both the phenomenal safety of MSRs and their potential to help solve so many of today’s energy-related problems, from climate change to energy poverty to the intermittency of wind and solar power.
In fact, MSRs can operate so safely, they may alleviate public fears about nuclear energy. Before looking at the potential of MSRs, though, it is useful to first take a high-level look at what they are and how they work.
What is a molten salt reactor?
A molten salt reactor (MSR) is a type of nuclear reactor that uses a liquid mixture of salts as both fuel and coolant, instead of the solid fuel rods used in conventional nuclear reactors.
This mixture is heated to high temperatures, causing the atomic nuclei within the fuel to split and release energy. This energy is then harnessed to produce electricity.
How a molten salt reactor works
Using liquid fuel provides many advantages in safety and simplicity of design.

The figure above shows one type of MSR design. As shown towards the left, the reactor contains “fuel salt”, which is fuel (such as uranium-235) dissolved in a mixture of molten fluoride salts.
After a fission chain reaction starts in the reactor, the rate of fission stabilizes once the fuel salt reaches around 700 degrees Celsius. If the reactor gets hotter than 700 degrees, the resulting expansion of the fuel salt pushes some of the fuel into the circulation loop. This, in turn, decreases the fission rate (since fission cannot be maintained in the loop), causing the fuel to cool.
Unlike conventional reactors, the rate of fission in an MSR is inherently stable. Nonetheless, should the fuel salt become too hot to operate safely, a freeze plug (made of salts kept solid by a cooling fan) below the reactor will melt and the liquid content of the reactor will flow down into emergency dump tanks where it cannot continue to fission, thus allowing it to cool safely.
The control rods at the top of the reactor provide further control of the rate of fission by absorbing neutrons that might otherwise cause a fission reaction. A test in the 1960s showed that an MSR can continue to run safely without operator intervention even after the intentional removal of a control rod during full operation.
The fuel salt is circulated through a heat exchanger where it is cooled by another molten salt loop that is free of radioactive fuel and fission products. The heat from this second loop can be used to do work, such as heating water to turn a steam turbine to generate electricity.
The fuel salt is also circulated through a chemical processing plant. This plant is used to both remove undesired fission products and add more fuel to the reactor.
The difference between molten salt reactors and conventional nuclear reactors
So, how do MSRs differ from the nuclear reactors we’re familiar with? The key difference lies in the state of the fuel and the way the heat is transferred.
In conventional reactors, the fuel is solid and the heat generated by nuclear fission is transferred to a coolant, often water. This coolant is then used to produce steam, which drives a turbine and generates electricity.
In contrast, MSRs use a liquid fuel, which doubles as the coolant. This means the heat transfer process is more efficient, as there’s no need for a separate coolant. Additionally, because the fuel is liquid, it can be continuously circulated and filtered, allowing for the removal of fission products and the addition of fresh fuel.
Are molten salt reactors safe?
Safety is a paramount concern when it comes to nuclear energy. The good news is that MSRs have several safety advantages over conventional reactors.
MSRs are walk-away safe. They cannot melt down like conventional reactors because they are, by design, already molten. An operator cannot even force an MSR to overheat. If for some reason an MSR were to overheat, the heat would melt a freeze-plug at the bottom of the reactor vessel and the liquid fuel salts would drain into the emergency cooling tanks where they would cool and solidify. No operator interaction nor even emergency backup power is needed for this to happen.
Even a human-engineered breach (such as a terrorist attack) of an MSR cannot cause any significant release of radioactivity. The fuel salts for MSRs work at normal atmospheric pressure, so a breach of the reactor containment vessel would simply leak out the liquid fuel which would then solidify as it cooled.
By comparison, a breach of a conventional reactor leads to the highly pressurized and radioactive water coolant spewing into the atmosphere and potentially leaking into surrounding bodies of water. Additionally, radioactive byproducts of fission like iodine-131, cesium-134 and cesium-137 — such as those released into the atmosphere and ocean by the Fukushima meltdown — are physically bound to the hardened coolant and do not leave the reactor site.
Why molten salt reactors?
MSRs are a huge departure from the conventional reactors most people are familiar with. Key features include:
A solution to nuclear waste and stockpiles of plutonium
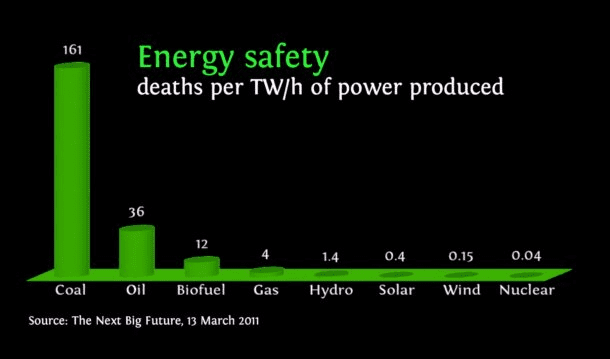
Conventional reactors use solid ceramic fuel rods containing enriched uranium. The fission of uranium in the fuel releases gases, such as xenon, which causes the fuel rods to crack. This cracking, in turn, makes it necessary to remove and replace the fuel rods well before most of the actinides (elements that remain radioactive for thousands of years) such as uranium have fissioned.
This is why nuclear waste is radioactive for a very long time.
However, the actinides that remain in the cracked fuel rods are still an excellent source of fuel for reactors. France, for example, recycles the waste instead of burying it so that these actinides can be placed in new fuel rods and used to make more electricity.
Because MSRs use liquid fuel, the release of gases simply bubbles up, typically to an off-gas unit in the coolant loop where it can be removed. Since the liquid fuel is unaffected by the releases of gas, the fuel can be left in the reactor until almost all the actinides are fissioned, leaving only elements that are radioactive for a relatively short time (300 years or less).
The result is that MSRs have no long-term issue with regard to nuclear waste.
MSRs can also be used to dispose of current stockpiles of nuclear waste by using those stockpiles as fuel. Even stockpiles of plutonium can be disposed of this way. In fact, conventional reactors typically use only 3-to-5% of the available energy in their fuel rods before the fuel rods must be replaced due to cracking. MSRs can use up most of the rest of the available fuel in these rods to make electricity.
Note: The reason that conventional reactors can’t use up all actinides in their fuel rods is a bit more complex than what is described above. The neutrons in conventional reactors only move fast enough to cause enriched uranium to fission. Fissioning most of the actinides requires much faster-moving neutrons, which can be achieved in both MSRs and solid-fuel reactors, such as the GE Hitachi PRISM.
Abundant energy that is much cheaper than coal
How do we get all 8 billion people on the planet to agree to drastically cut their CO2 emissions? The answer: make it in their immediate self-interest by providing cheap CO2-free energy — energy cheaper than they can get by burning coal.
MSRs can be made cheaply because they are simple compared to conventional reactors that have large, pressurized containment domes and many engineered (and not inherent), redundant safety systems.
Having far fewer parts than conventional reactors, MSRs are inherently cheaper. This simplicity also allows MSRs to be small, which in turn makes them ideal for factory-based mass production (unlike conventional reactors). The cost efficiencies associated with mass production further drive down the cost and can make the ramp-up of nuclear power much faster.
Load following solar and wind power
A significant limitation of solar and wind power is their intermittency and unreliability. Currently, these issues are dealt with in the U.S. by quickly firing-up natural gas plants to load follow solar and wind power.
In other words, gas plants must ramp up quickly when power from wind and sun is scarce, and ramp down quickly when the sun is shining or the wind is blowing. Unfortunately, this is an inefficient way to burn natural gas, which can result in almost as much CO2 output from gas plants ramping up and down as from when they simply run continuously.
And, of course, continued use of natural gas requires continued fracking. Many hope that a grid-level energy storage technology will someday negate the need to use natural gas plants, but no economic energy storage is on the horizon.
Unlike conventional nuclear reactors, the characteristics of MSRs make them good candidates for CO2-free load following of solar and wind power.
This is because slowing down nuclear reactions results in an increased release of xenon gas. When conventional reactors do this, they must wait several days to restart while the xenon gas decays. This extra xenon is not a problem for MSRs because of their off-gas system, which allows immediate removal of xenon; hence, no delay is needed after ramping up or down an MSR.
Note that conventional reactors can be designed to load follow, but typically haven’t been for economic reasons (more profit can be made by running conventional reactors at full power for base load applications).
Abundant energy for millions of years
Although it is sometimes claimed that nuclear power is not sustainable, the truth is that there is enough nuclear fuel on Earth to provide humanity with abundant energy for millions of years.
MSRs can run on uranium and existing stockpiles of plutonium and nuclear waste. A variant of an MSR, a liquid fluoride thorium reactor (LFTR), will be able to use abundant thorium as a fuel.
In addition, breeder reactors (which include some types of MSRs) make it possible to use uranium-238 as fuel, which makes up 93.3% of all natural uranium. Conventional reactors use only uranium-235, which makes up a mere 0.7% of natural uranium.
Replaces fossil fuels where wind and solar are problematic
MSR technology has potential far beyond generating electricity cheaply and without emitting CO2. For example, MSRs could be used to replace fossil fuels for high-heat industrial processes such as water desalination and the production of cement and aluminum.
In the U.S., industrial processes account for a little over 5% of greenhouse gases. MSRs can even provide high heat for cheap production of feedstock for synthetic, CO2-free liquid fuels.
MSRs could also be used to power large container ships, which currently run on diesel. The 15 largest of these ships produce as much air pollution every day as all of the cars on the planet.
Molten salt reactors can’t be used to make nuclear weapons
No nuclear reactor can be made proliferation-proof, but MSRs have some significant advantages for proliferation resistance. First, the waste from MSRs is not useful for use in nuclear weapons since MSRs fission almost all actinides.
Second, MSRs can use up existing stockpiles of nuclear waste from conventional reactors as well as existing stockpiles of plutonium, making these materials unavailable for use in nuclear weapons.
Examples of molten salt reactors
MSRs aren’t just theoretical constructs; they’re being developed and tested around the world. For instance, the Shanghai Institute of Applied Physics is working on a thorium-based MSR, with the aim of having a fully operational reactor by 2030.
In the United States, a company called Terrestrial Energy is developing an Integral Molten Salt Reactor (IMSR). This design aims to be safer and more cost-effective than traditional reactors, with a smaller footprint and the ability to be mass-produced.
A Very Brief History of MSR Technology

MSRs were first developed in the U.S. in the 1950s for use in a nuclear-powered aircraft bomber (the idea being that the bomber could remain in the air indefinitely). Though a small experimental reactor ran successfully, the program was canceled when it became clear that in-air refueling of bombers was viable.
Under the supervision of Alvin Weinberg in the 1960s, Oak Ridge National Laboratory built an experimental MSR that ran successfully for four years. Weinberg realized early on that MSRs were the ideal type of reactor for civilian use because they cannot melt down. He was eventually fired by the Nixon administration for this advocacy.

In the 2000s, then NASA engineer Kirk Sorenson, who was tasked with figuring out how to power a station on the moon, found that MSRs were the best solution. He also realized that MSRs are a great solution on Earth too. His tireless advocacy for MSRs has generated much interest.
Conclusion
The Intergovernmental Panel on Climate Change, the International Energy Agency, and the United Nations, and even over 70% of climate scientists agree that we must ramp up nuclear power if we are going to succeed in dealing with climate change.
Because of its exceptional safety and low cost, perhaps MSR technology is a nuclear technology that almost everyone can embrace.