Engines are machines that convert a source of energy into physical work. If you need something to move around, an engine is just the thing to slap onto it. But not all engines are made the same, and different types of engines definitely don’t work the same.

Probably the most intuitive way to differentiate between them is the type of energy each engine uses for power.
- Thermal engines
- Internal combustion engines (IC engines)
- External combustion engines (EC engines)
- Reaction engines
- Electrical engines
- Physical engines
Thermal engines
In the broadest definition possible, these engines require a source of heat to convert into motion. Depending on how they generate said heat, these can be combustive (that burn stuff) or non-combustive engines. They function either through direct combustion of a propellant or through the transformation of a fluid to generate work. As such, most thermal engines also see some overlap with chemical drive systems. They can be airbreathing engines (that take oxidizer such as oxygen from the atmosphere) or non-airbreathing engines (that have oxidizers chemically tied in the fuel).
Internal combustion engines
Internal combustion engines (IC engines) are pretty ubiquitous today. They power cars, lawnmowers, helicopters, and so on. The biggest IC engine can generate 109,000 HP to power a ship that moves 20,000 containers. IC engines derive energy from fuel burned inside a specialized area of the system called a combustion chamber. The process of combustion generates reaction products (exhaust) with a much greater total volume than that of the reactants combined (fuel and oxidizer). This expansion is the actual bread and butter of IC engines — this is what actually provides the motion. Heat is only a byproduct of combustion and represents a wasted part of the fuel’s energy store, because it doesn’t actually provide any physical work.

Image credits NASA / Glenn Research Center.
IC engines are differentiated by the number of ‘strokes’ or cycles each piston makes for a full rotation of the crankshaft. Most common today are four-stroke engines, which break down the combustion reaction in four steps:
- Induction or injection of a fuel-air mix (the carburate) into the combustion chamber.
- Compression of the mix.
- Ignition by a spark plug or compression — fuel goes boom.
- Emission of the exhaust.
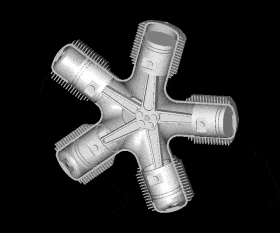
Image credits Duk / Wikimedia.
For every step, a 4-stroke piston is alternatively pushed down or back up. Ignition is the only step where work is generated in the engine, so for all other steps, each piston relies on energy from external sources (the other pistons, an electric starter, manual cranking, or the crankshaft’s inertia) to move. That’s why you have to pull the chord on your lawnmower, and why your car needs a working battery to start running.
Other criteria for differentiating IC engines are the type of fuel used, the number of cylinders, total displacement (internal volume of cylinders), distribution of cylinders (inline, radial, V-engines, etc.), as well as power and power-to-weight output.
External combustion engines
External combustion engines (EC engines) keep the fuel and exhaust products separately — they burn fuel in one chamber and heat the working fluid inside the engine through a heat exchanger or the engine’s wall. That grand daddy-o of the Industrial Revolution, the steam engine, falls into this category.
In some respects, EC engines function similarly to their IC counterparts — they both require heat which is obtained by burning stuff. There are, however, several differences as well.
EC engines use fluids that undergo thermal dilation-contraction or a shift in phase, but whose chemical composition remains unaltered. The fluid used can either be gaseous (as in the Stirling engine), liquid (the Organic Rankine cycle engine), or undergo a change of phase (as in the steam engine) — for IC engines, the fluid is almost universally a liquid fuel and air mixture that combusts (changes its chemical composition). Finally, the engines can either exhaust the fluid after use like IC engines do (open-cycle engines) or continually use the same fluid (closed-cycle engines).
Surprisingly, the first steam engines to see industrial use generated work by creating a vacuum rather than pressure. Called ‘atmospheric engines’, these were ponderous machines and highly fuel inefficient. In time, steam engines took on the form and characteristics we expect to see from engines today and became more efficient — with reciprocating steam engines introducing the piston system (still in use by IC engines today) or compound engine systems that re-used the fluid in cylinders at decreasing pressures to generate extra ‘oomph’.
Today, steam engines have fallen out of widespread use: they’re heavy, bulky things, have a much lower fuel efficiency and power-to-weight ratio than IC engines, and can’t change output as quickly. But if you’re not bothered by their weight, size, and need a steady supply of work, they’re awesome. As such, EC is currently employed with great success as steam turbine engines for naval operations and power plants.
Nuclear power applications have the distinction of being called non-combustive or external thermal engines since they operate on the same principles of EC engines but don’t derive their power from combustion.
Reaction engines
Reaction engines, colloquially known as jet engines, generate thrust by expelling reactionary mass. The basic principle behind a reactionary engine is Newton’s Third Law — basically, if you blow something with enough force through the back end of the engine, it will push the front end forward. And jet engines are really good at doing that.

Image credits thund3rbolt / Imgur.
The things we usually refer to as a ‘jet’ engine, the ones strapped to a Boeing passenger plane, are strictly speaking airbreathing jet engines and fall under the turbine-powered class of engines. Ramjet engines, which are usually considered simpler and more reliable as they contain fewer (up to none) moving parts, are also airbreathing jet engines but fall into the ram-powered class. The difference between the two is that ramjets rely on sheer speed to feed air into the engine, whereas turbojets use turbines to draw in and compress air into the combustion chamber. Beyond that, they function largely the same.
In turbojets, air is drawn into the engine chamber and compressed by a rotating turbine. Ramjets draw and compress it by going really fast. Inside the engine, it’s mixed with high-power fuel and ignited. When you concentrate air (and thus oxygen), mix it up with a lot of fuel and detonate it (thus generating exhaust and thermally expanding all the gas), you get a reactionary product that has a huge volume compared to the air drawn in. The only place all this mass of gasses can go through is to the back end of the engine, which it does with extreme force. On the way there, it powers the turbine, drawing in more air and sustaining the reaction. And just to add insult to injury, at the back end of the engine there’s a propelling nozzle.

This piece of hardware forces all the gas to pass through an even smaller space than it initially came in by — thus further accelerating it into ‘a jet’ of matter. The exhaust exits the engine at incredible speeds, up to three times the speed of sound, pushing the plane forward.
Non-airbreathing jet engines, or rocket engines, function just like jet engines without the front bit — because they don’t need external material to sustain combustion. We can use them in space because they have all the oxidizer they need, packed up in the fuel. They’re one of the few engine types to consistently use solid fuels.
Heat engines can be ridiculously big, or adorably small. But what if all you have is a socket, and you need to power your stuff? Well, in that case, you need:
Electrical engines
Ah yes, the clean gang. There are three types of classical electrical engines: magnetic, piezoelectric, and electrostatic.

The magnetic one, like the battery there, is the most commonly used of the three. It relies on the interaction between a magnetic field and electrical flow to generate work. It functions on the same principle a dynamo uses to generate electricity, but in reverse. In fact, you can generate a bit of electrical power if you hand crank an electrical-magnetic motor.
To create a magnetic motor you need some magnets and a wound conductor. When an electrical current is applied to the winding, it induces a magnetic field that interacts with the magnet to create rotation. It’s important to keep these two elements separated, so electrical motors have two major components: the stator, which is the engine’s outer part and remains immobile, a rotor that spins inside it. The two are separated by an air gap. Usually, magnets are embedded into the stator and the conductor is wound around the rotor, but the two are interchangeable. Magnetic motors are also equipped with a commutator to shift electrical flow and modulate the induced magnetic field as the rotor is spinning to maintain rotation.
Piezoelectric drives are types of engines that harness some materials’ property of generating ultrasonic vibrations when subjected to a flow of electricity in order to create work. Electrostatic engines use like-charges to repulse each other and generate rotation in the rotor. Since the first uses expensive materials and the second requires comparatively high voltages to run, they’re not as common as magnetic drives.
Classical electrical engines have some of the highest energy efficiency of all the engines out there, converting up to 90% of energy into work.
Ion drives
Ion drives are kind of a mix between a jet engine and an electrostatic one. This class of drives accelerates ions (plasma) using an electrical charge to generate propulsion. They don’t function if there are ions already around the craft, so they’re useless outside of the vacuum of space.
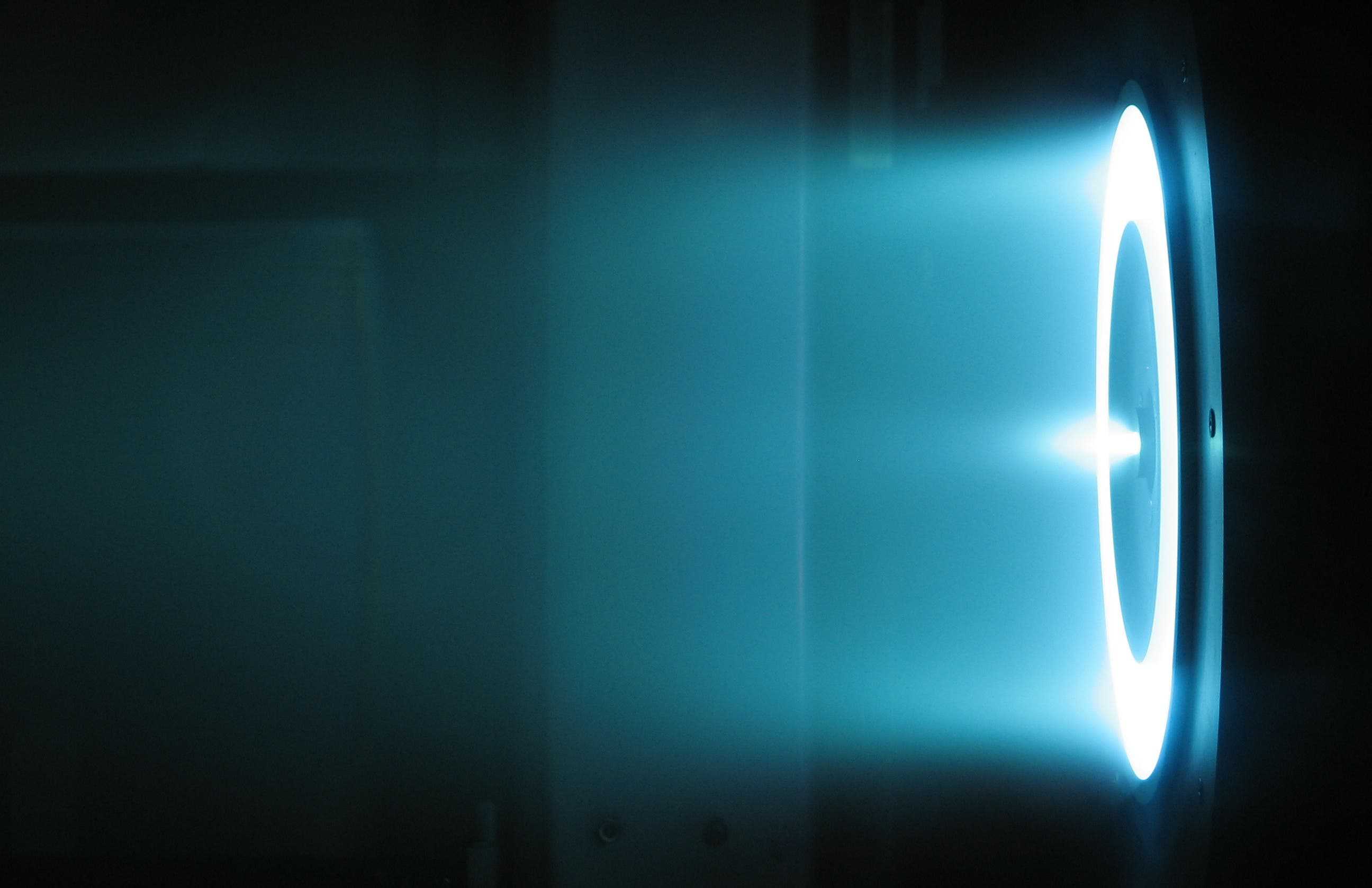
Image credits NASA / JPL-Caltech.
They also have a very limited power output. However, since they only use electricity and individual particles of gas as fuel, they’ve been studied extensively for use in spaceships. Deep Space 1 and Dawn have successfully used ion drives. Still, the technology seems best suited for small craft and satellites since the electron trail left by these drives negatively impacts their overall performance.
EM/Cannae drives
EM/Cannae drives use electromagnetic radiation contained in a microwave cavity to generate trust. It’s probably the most peculiar among all types of engines. It’s even been referred to as the ‘impossible’ drive since it’s a nonreactionary drive — meaning it doesn’t produce any discharge to generate thrust, seemingly bypassing the Third Law.
“Instead of fuel, it uses microwaves bouncing off a carefully tuned set of reflectors to achieve small amounts of force and therefore achieve propellant-free thrust,” Andrei reported on the drive.
There was a lot of debate on whether this type of engine actually works or not, but NASA tests have confirmed it’s functionally sound. It’s even getting an upgrade in the future. Since it uses only electrical power to generate thrust, albeit in tiny amounts, it seems to be the best-suited drive for space exploration.
But that’s in the future. Let’s take a look at how it all started. Let’s take a look at:
Physical engines
These engines rely on stored mechanical energy to function. Clockwork engines, pneumatic, and hydraulic engines are all physical drives.

Image credits Musée national de la Marine.
They’re not terribly efficient. They usually can’t call upon large energy reserves either. Clockwork engines for example store elastic energy in springs, and need to be wound each day. Pneumatic and hydraulic types of engines have to carry hefty tubes of compressed fluids around, which generally don’t last very long. For example, the Plongeur, the world’s first mechanically powered submarine built in France between 1860 and 1863, carried a reciprocating air engine supplied by 23 tanks at 12.5 bars. They took up a huge amount of space (153 cubic m / 5,403 cubic ft) and were only enough to power the craft for 5 nautical miles (9 km / 5.6 mi) at 4 knots.
Still, physical drives were probably the first ever used. Catapults, trebuchets, or battering rams all rely on this type of engines. So too are man or beast powered cranes — all of which have been in use long before any other kind of engines.
This is by no means a complete list of all the engines man has made. Not to mention that biology has produced drives too — and they’re among the most efficient we’ve ever seen. But if you read all of this, I’m pretty sure yours are running out of fuel by this point. So rest, relax, and the next time you come across an engine, get your hands and your nose all greased up exploring through it — we’ve told you the basics.