The Sun is a ball of nuclear plasma so large its own weight keeps it from exploding. Very cool, but also quite hot. However, the Sun has a complicated interior structure, and surprisingly large temperature variations, both on and under its surface. Today, we’re going to look at why this is, and how we know.

A lot of the things happening on Earth are, ultimately, fueled by energy from the Sun. We see that energy as sunshine, feel it hot on our skin on a clear day. It drives winds, and it powers rain cycles. Almost all life on Earth is fed by plants capturing sunlight. It’s very fortunate for us, then, that the Sun produces a monumental amount of energy. Just a fraction of its output reaches our planet, since a lot of it is lost in transit, reflected, or radiates away from Earth and into the void. Even so, it’s much more than we’d know what to do with, and only about 1% of it is enough to keep all the plants on Earth alive.
Energy is never lost or created, but transformed from one ‘flavor’ into another. Still, not all of them are equal, and heat seems to be the baseline that all others eventually degrade into. The Sun, therefore, gets quite hot.
Just how hot?
It depends on a lot of factors — mostly on exactly where you’re taking the measurement. There’s a lot of variation here.
First off is the Sun’s core. Here is where the fusion reaction that drives the star actually takes place. Due to the sheer mass of gas pressing down on the core, ambient pressure here is immense. Temperatures, too, are extremely high, due to how compressed everything gets. This is ideal, because such extreme conditions are needed for fusion to take place. To the best of our knowledge, temperatures at the core of the Sun can reach in excess of 15 million °C (27 million °F), which is a lot.
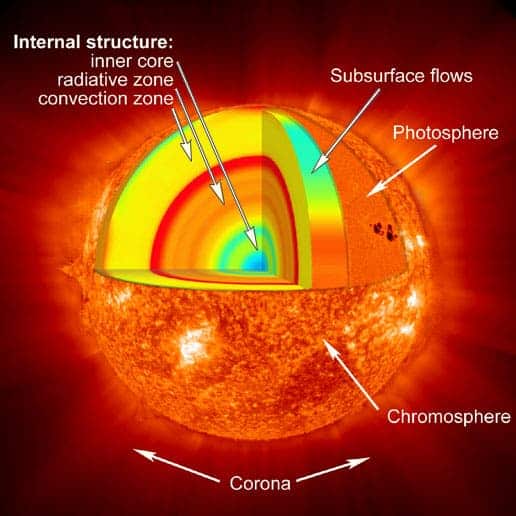
The next layer of the star is its ‘radiative zone’. Energy from the core moves out to this area, carried by bodies of superheated, ionized atoms, where it becomes trapped. It spends up to 1 million years here, before finally managing to escape the strong gravity and electromagnetic fields and reach the convective zone. This zone represents the upper layer of the Sun’s core, and temperatures here are believed to be around 2 million °C (3.5 million °F).
Hot plasma from this convective zone can bubble up towards the surface of the Sun. The next layer it encounters is the photosphere, which is about 5,500 °C (10,000 °F), significantly cooler compared to the previous layer. It is here that the radiation produced inside the star can first be perceived as light by an outside observer. A photosphere (‘sphere of light’ in ancient Greek) is defined as the deepest region of a light-emitting body that is still transparent to photons of certain wavelengths. In other words, the photosphere starts where the Sun’s plasma becomes transparent enough for light to be able to escape it.
However, the photosphere is not uniform. Areas of intense electromagnetic activity produce sunspots, which are darker and cooler than their surroundings; temperatures in the center of a sunspot can drop to lows of 4,000 °C (7,300 °F).
The next layer, the chromosphere (‘sphere of colors’ in ancient Greek), is a tad cooler, at about 4,320 °C (7,800 °F) on average. Light from this layer is thus dimmer, and we don’t usually see it. But, during a solar eclipse (when the moon covers the sun), this is the really fancy bit you see around its outline, the red rim surrounding the Sun. This color is emitted by the high content of hydrogen gas in the chromosphere.
Coronal loops, bands of ionized gas trapped by the Sun’s churning magnetic fields, photographed by NASA’ TRACE. They are often associated with sunspots. Image credits NASA. Our first image of a sunspot, taken on January 28, 2020 by the NSF’s Inouye Solar Telescope’s Wave Front Correction. Image credits NSO / AURA / NSF.
In relative terms, temperatures in the photo- and chromatosphere aren’t that high — a candle, for example, burns at around 1,000 °C (1,800 °F). We know these two layers exist because their relatively mellow conditions allow for simple molecules such as water and carbon monoxide to survive, and we’ve picked up on their spectral emissions.
Lastly, there is the corona — the Sun’s crown. A bit unexpectedly, temperatures shoot back up in this layer, despite it being the farthest away from the core. In fact, it has average temperatures at the same order of magnitude as the core, although they are still lower. These range between 1 million °C and 10 million °C (roughly 1.7 – 17 million °F), according to the National Solar Observatory (NSO). The corona and chromatosphere are kept separated from this layer by a transition zone of highly-ionized helium atoms. This is less of a hard boundary and more of a chaotic, ever-churning sea of clouds. The corona might be so hot due to ‘nanoflares‘, but we’re still unsure.
Beyond the corona lies the Sun’s extended atmosphere, the heliosphere, which is less of a layer per se and more of an area of influence that the Sun exerts. While emissions such as solar winds or flares can send super-heated, charged particles flying off from a star into its heliosphere, this is much cooler than the layers we’ve discussed previously. The main component of the heliosphere is magnetic, and it has a key part to play in forming the Sun’s shield around our solar system.
How do we know how hot it is?

Sticking a thermometer in the Sun is, understandably, a bit tricky. So we’ve had to rely on indirect methods of measurement to tell exactly how hot it can become.
If you put a kettle on to boil, you’ll be able to feel heat coming from the water even after you take it off the stove. How much of it you will perceive depends a lot on how close you hold your hand to the water. This process relies on the radiative properties of electromagnetic energy. To keep it simple, particles start moving when they heat up, and this motion generates thermal radiation; infrared cameras pick up on this kind of radiation, for example. Our senses perceive it as heat.
One of the simplest and also least accurate ways of assessing the Sun’s temperature is our own senses. Sunlight can be really hot on a clear day. Considering the star is around 149 million kilometers away, it must be outputting a lot of thermal energy for it to reach all the way here. Still, researchers like numbers, especially accurate ones, so they did develop several other means of gauging the star’s temperature.
One approach uses the link between heat and the light coming from a body — because in physics, unlike life, being hotter automatically makes you brighter, too.
Thermal radiation is a kind of electromagnetic radiation, but so is light. In very broad terms, as long as the cause is temperature, the brighter an object glows, the hotter it is. Irons heating in a forge are a good example. The hue can help us determine this temperature exactly. Red light is cooler than yellow light which is cooler than blue, and so on. A yellow-flamed candle burns colder than the blue flames on your stove.

That’s the working principle. In practice it becomes more complicated since the Sun doesn’t output a single type (wavelength) of light, but a whole cocktail of wavelengths that mix and interact to create the final, white light we perceive. We use a device called a spectrograph to tease these colors apart into individual wavelengths. They work much like raindrops do when creating rainbows.
Once we break down sunlight like this, we can look at each individual color (wavelength) of light and determine what temperature is associated with it. Every wavelength carries different amounts of energy, so the final step is to average out their temperatures to determine the final ‘product’. Think of it like determining the energy level of the middle-most color by looking at each individual color present in sunlight.
Sunlight can also be used to determine the chemical composition of our star. Every stellar body emits light across multiple wavelengths. But these ’emission spectra’ also show very small, generally well-defined gaps, tight wavelength intervals where no light is emitted (or where light is being absorbed). This comes down to how atoms interact with radiation but, suffice to say, these gaps are extremely reliable signatures of certain elements. As long as you understand the trace each of them leaves on the emission spectrum, you can determine a body’s composition from the light it emits. We call these traces Fraunhofer lines.
Our Sun’s emission spectrum, with its Fraunhofer lines in black, simplified. Every line showcases a tiny wavelength interval that’s missing from the final light. Image via Wikimedia. A high-resolution version of the spectrum of our Sun. Image credits N.A.Sharp, NOAO / NSO/ Kitt Peak FTS / AURA / NSF.
Which extinction lines are present, as well as how well-defined they are, are influenced by temperature. As such, this step can help determine both the composition and temperature of a star.
Still, we said earlier that the photosphere is a hard boundary in regards to our perception of light — we can’t see below this limit. Spectroscopy, then, as well as other optical methods, can only help us determine temperatures down to this layer. At the same time, even if the corona is much hotter, it’s also significantly less bright than the photosphere, so it has a very small contribution to this type of measurement.
As for anything deeper than the photosphere? That’s more theoretical. It’s not pulled out of our assumptions, but it’s still a theoretical estimate. Temperature conditions inside the Sun are based on the idea that it is in a state of hydrostatic equilibrium. That is, that its gravity (inward pressure) and expansive (outward pressure) generated by nuclear fusion at the core cancel each other out. If they wouldn’t, the star would blow up or turn black holey, so it’s a solid starting point.
If you combine this with chemical readings from spectroscopy, and estimates of the star’s mass (also calculated or obtained indirectly), you can determine what temperatures should be at the core to keep it all stable. We also know from our efforts at making fusion happen on Earth that humongous pressures and temperatures are needed to convince hydrogen atoms to merge; the Sun does that on a monumental scale, every second.
This is all based on tried-tested-and-true methods and theorems regarding natural processes, so they are reliable, but they’re still just estimates. If you’re the kind of person that needs exact measurements and figures, talking about the Sun might not be the best hobby for you. But, if it makes you find a way to go there and actually stick a thermometer in the thing so we can all find out, I won’t complain.