The term was first used in 2006 to describe celestial bodies that were comparable in size to Pluto. Since then it has taken on a broader usage, with the IAU (International Astronomers’ Union) currently recognizing five bodies in the Solar System as being dwarf planets — and an extra six more await a decision.

Image credits ESO / L. Calçada and Nick Risinger.
However, since its addition to the old classification system (which held that nine planets were orbiting the Sun), the term ‘dwarf planet’ has caused quite a lot of confusion (and controversy). So let’s see what it’s meant to represent and whether Pluto does actually deserve to be called as such.
Planet-ish.
A dwarf planet is “(a) in orbit around the Sun [or another star], (b) has sufficient mass for its self-gravity to overcome rigid body forces so that it assumes a hydrostatic equilibrium (nearly round) shape, (c) has not cleared the neighborhood around its orbit, and (d) is not a satellite,” according to the IAU’s RESOLUTION B5 on the Definition of a Planet in the Solar System.
In essence, that definition says that dwarf planets are objects in stable orbit around a star that are massive enough to maintain a round shape but not massive enough to clear all other material out of their orbit, and that they don’t orbit another planet.
It’s basically these last two points that differentiate a dwarf planet from a regular one and from moons. Full-blown planets always clear their orbits, according to the current definition, but neither they nor dwarf planets can orbit another planet — only moons do that.
There are currently five dwarf planets recognized as such by the IAU in the Solar System: Pluto, Ceres, Eris, Makemake, and Haumea. There is still some debate whether Eris, Makemake, or Haumea fit the bill, however, as our observations of these bodies are still far from perfect. Other nearby contenders include Orcus, 2002 MS4, Salacia, Quaoar, 2007 OR10, and Sedna; the IAU further decided that Trans-Neptunian Objects (TNOs) with an absolute magnitude higher than +1 and a diameter of 838 km or more are to be considered dwarf planets until more data can be gathered on their nature.
On the matter of shape

Image credits NASA / JPL.
The roundedness criteria is actually pretty central in how we think about planets. Roundedness in the case of stellar objects is largely the product of gravity. Planets become round (sorry, Flat Earthers) when their gravity becomes strong enough to plastically deform their surfaces and to overpower all other forces affecting them. Strong gravity is necessary to impart a rounded shape to a planet by flattening high-elevation areas and filling in low-lying ones.
It’s a pretty reliable indicator of planethood, all things considered. Asteroids or comets are small and don’t have the mass to round themselves out; their final shape is the product of outside forces acting on the body. Centrifugal forces generated by rotation, friction, impacts, or the tidal pull of other bodies are what create their irregular shapes.
Those bodies for which gravity is a significant but not dominant factor morph into spheroid (sphere-like) shapes. This is a bit of a middle-ground between the shape gravity works towards and the irregular mess promoted by outside forces.
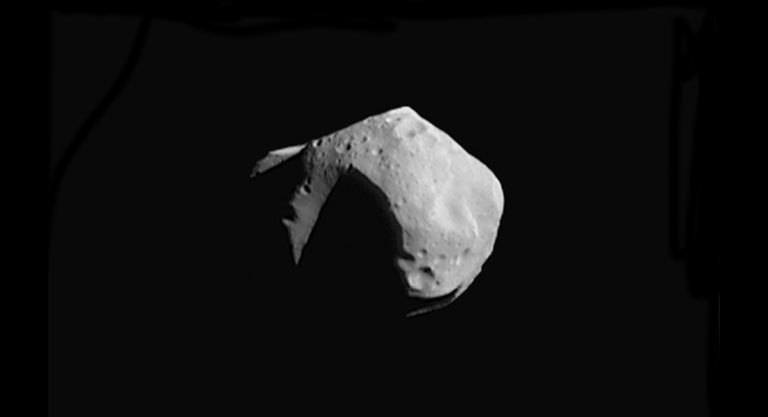
Image credits NASA / JPL.
An object with high gravity will come as close to a perfect sphere as possible, reaching ‘hydrostatic equilibrium‘. To illustrate, the Earth isn’t perfectly round — it’s an oblate spheroid. This is due to the planet’s rotation around its own axis, which generates centrifugal forces that pull on the equator, making the Earth look a bit like a sphere you pressed down on. The faster a body rotates, the more deformation it will experience around its equator. The dwarf planet Haumea, for example, is almost twice as long at its equator as it is at the poles.
Tidal forces are the gravitational pull of other planets and/or celestial bodies on an object. This helps deform it (on Earth we see this as the waxing and waning of the tide) and can tidally-lock an object in relation to another. The moon is tidally locked to Earth; no matter when you look at it, it’s always showing the same hemisphere.

Image credits Jin Zan via Wikimedia.
The IAU’s definition uses shape instead of mass because particularities of a given celestial body, such as its chemical composition, also have a part to play in their overall shape. Mass alone isn’t a reliable indicator of a body’s overall characteristics. Water ice, for example, is much more easily deformed by gravity than a chunk of solid rock.
On the matter of its neighborhood
A celestial body’s ability to remove all smaller ones along its orbit is also known as ‘orbital dominance’. Planets have virtually complete orbital dominance through collision, capture, or other interaction with other space-born bodies they come into contact with; dwarf planets do not.
Orbital dominance once again ties into a body’s mass, which dictates its gravitational force (do keep in mind that gravitational pull is inversely proportional to the squared distance between two bodies).
An easy way to think about this factor is that planets have enough mass to ‘overpower’ all other bodies of similar size in the volume of space they transit. Dwarf planets, being smaller, need to share their space with other chunks of matter.

Image credits NASA / JPL.
This is the most contentious point in the definition. For one, an argument can be made that a planet’s orbit is never fully cleared as objects come and go through space. Secondly, there’s also the question of how can we reliably say that a planet did in fact clear its orbital neighborhood; and also, for that matter, where does a planet’s neighborhood end? The IAU doesn’t put any actual figures on its definition of dwarf planets. The definition can thus be seen as more of a theoretical guideline than a hard criterion; it has drawn criticism for this fact.
“In no other branch of science am I familiar with something that absurd,” said New Horizons principal investigator Alan Stern for Space.com in 2011. “A river is a river, independent of whether there are other rivers nearby.”
“In science, we call things what they are based on their attributes, not what they’re next to.”
Stern says that Earth, Mars, Jupiter, and Neptune have not fully cleared their orbital zones either, but we still call them planets. Some 10,000 near-Earth asteroids orbit the Sun alongside our planet, he explains. Jupiter drags some 100,000 Trojan asteroids on its way through space, Stern adds. The main criticism leveled at his approach is that these planets completely control the other asteroids and bodies within their orbit via gravitational pull.
The debate is especially heated because Pluto’s status as a planet hinges on this particular point of the IAU’s definition. Pluto is gravitationally dominated by Neptune, which constrains its orbit. It also has to share its neighborhood with several objects in the Kuiper belt of similar size, effectively dooming it to a dwarf planet status under the current definition.
It’s also relevant for exoplanets. Our current equipment and techniques can’t directly determine whether a planet very far away has cleared its orbit. Here, however, the IAU has taken some steps to clarify matters: it established a separate working definition for extrasolar planets in 2001, and decided that the minimum size and mass requirements for planets in the Solar System apply to exoplanets as well.
“As new claims are made in the future, the WGESP [Working Group on Extrasolar Planets] will weigh their individual merits and circumstances and will try to fit the new objects into the WGESP definition of a “planet”, revising this definition as necessary,” the statement reads.
“This is a gradualist approach with an evolving definition, guided by the observations that will decide all in the end.”
The main current issues with the IAU’s classification system is that while it’s easy to understand, it doesn’t really withstand impact with reality on the ground. Space is a big place and it doesn’t abide by simple rules you can fit in a four-point list.
However, we’re still in a very early stage of space exploration. The exact difference between planets, dwarf planets, and moons is pretty inconsequential to our lives, even if it does rile up the spirits. As our reach into space extends, such classifications will become more important. But our ability to clearly define the multitude of shapes, sizes, and types of matter we’ll find in space will also be much better by then.