The Universe is filled with a near-uniform field of radiation, a fossil frozen into the fabric of spacetime by an event that happened shortly after the Big Bang. Made of photons from the Universe’s first light this ubiquitous radiation is known as the Cosmic Microwave Background (CMB).
And just as the paleontological study of fossils unlocks the secrets of how prehistoric animals existed, the lives they led, and how they evolved, this cosmic fossil reveals how the Universe has evolved and how the galaxies and stars were born.
The story of its discovery is one of good fortune, serendipity, and pigeon poo, and its current investigation involves highly sensitive cutting-edge technology and enterprising space missions.
“It’s impossible to overstate the importance of (this) discovery,” Mark Kamionkowski, professor of physics and astronomy at Johns Hopkins University, told NJ.Com. “The CMB has turned out to be a Rosetta Stone on which all the information we have about the origin of our universe is transcribed. Cosmology would still be in the Stone Age if it weren’t for the CMB.”
Let There Be Light: The Creation of the Cosmic Microwave Background
The story of the CMB begins shortly after the Big Bang–the initial period of rapid inflation experienced at the beginning of the Universe. Immediately after the Big Bang kick-started cosmic expansion the Universe was filled with a sea of hot plasma that consisted of fundamental particles like electrons, quarks, and gluons.
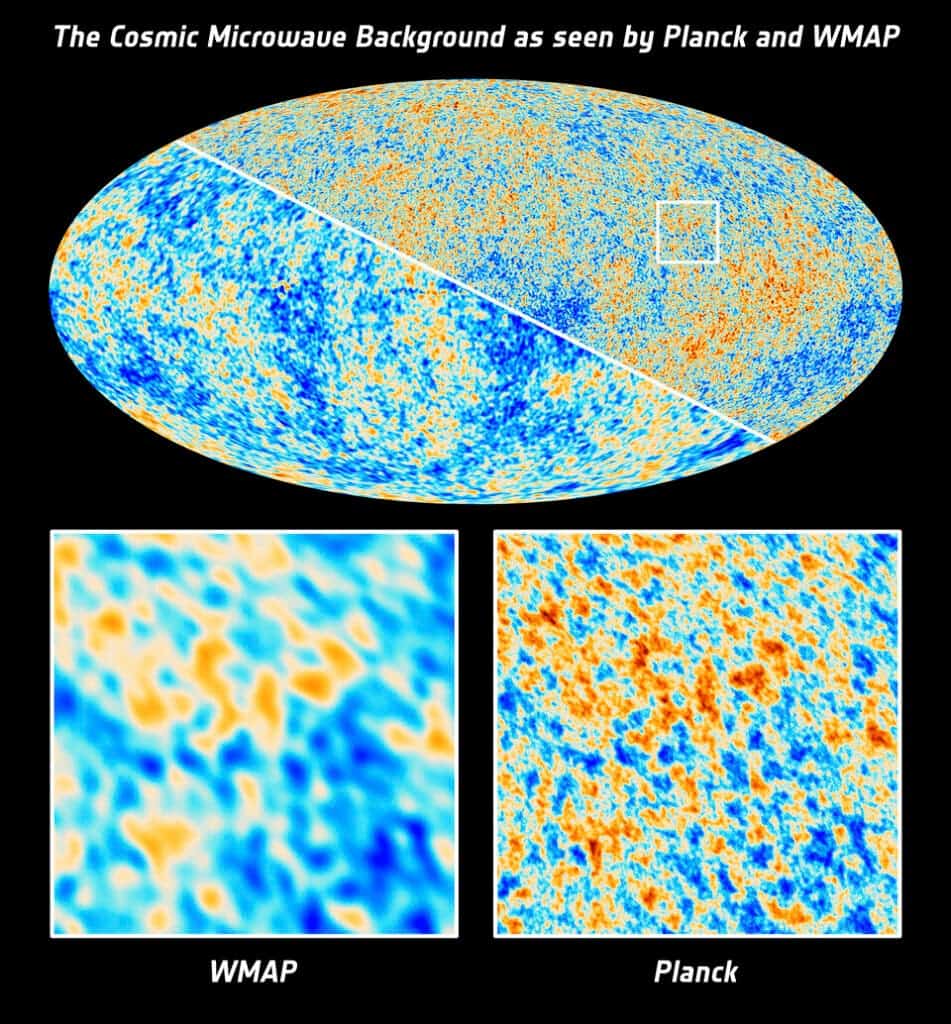
Weaved throughout this plasma soup were photons–the fundamental units of light– unable to travel because the plasma soup was dense enough to infinitely rebound them. This meant that the Universe was opaque. This would remain the case for around 380,000 years.
As the Universe expanded it also cooled. Eventually, the temperature had dropped low enough at about 3,000 K (2,700 degrees Celcius) to allow negatively charged electrons to bond–or recombine–with positively charged protons creating the first hydrogen atoms.
Known as the era of recombination, the reduction of free electrons at this point meant that photons were suddenly allowed to travel freely. This meant that the Universe was suddenly transparent.
This endless scattering between photons and matter had kept the Universe in a state called thermal equilibrium. The recombination era marked the point at which this equilibrium in temperature between matter and radiation ended as photons and particles of matter ‘decoupled.’
The CMB is a relic of this period composed of these photons that represent the Universe’s ‘first light.’ The radiation approaches an observer from a spherical surface surrounding them. The radius of the shell–called the ‘last scattering surface’–is the distance each photon has traveled since it was last scattered at the epoch of recombination.
For this reason, the CMB marks the furthest we could ever hope to investigate using light because beyond this point the Universe is opaque meaning this is effectively a cosmic horizon in terms of light.
As indicated above, the temperature of the photons when they decoupled from matter was 3000 K, but they would not hold this temperature. As the Universe continued to expand, the wavelengths of these photons were stretched–or redshifted– and as a result, they lost energy.
Currently, photons from the CMB have an apparent temperature of 2.735 K (-270ºC ), having reduced by a factor of 1,000 over around 13 billion years. But, as the Universe continues to expand CMB photons will cool even further. By the time the Universe is 3 times its current size, these photons will have dropped to a temperature of just 1 K.
Thus the CMB was frozen in the Universe as a cosmic fossil all that was left was for someone to find it.
Discovering Cosmic Microwave Background
A lot hinged on the discovery of the CMB, by the 1950s there were two families of theories as to the nature of the Universe. The Steady State Theory suggested by Hermann Bondi, Thomas Gold, and Fred Hoyle stated the Universe was homogeneous in space and time and had always been that way. In other words, the Universe existed in a ‘steady state.’
This concept had been seriously shaken in 1929 when Edwin Hubble discovered that distant cosmic objects were uniformly moving away. This suggested that the Universe was expanding. Some physicists had taken this idea further suggesting the cosmos had expanded from an infinitely dense point. This concept was dismissively labeled as ‘the Big Bang’ by Gamow.
The existence of the CMB was first theorized by physicists George Gamow, Ralph Alpher, and Robert Herman in the 1940s. The trio was studying the creation of the lightest elements–hydrogen, helium, and lithium–via nucleosynthesis.
The team came to the realization that to exist in the quantities they do today these light elements must have been synthesised in a hot and dense Universe. This meant, they thought, that radiation from this ‘hot Big Bang’ should have cooled to around 5 k and should still be detectable today.
Despite a good background in theory, the CMB would go undiscovered for a further two decades and when it was found it was completely by accident and by two extremely frustrated researchers.
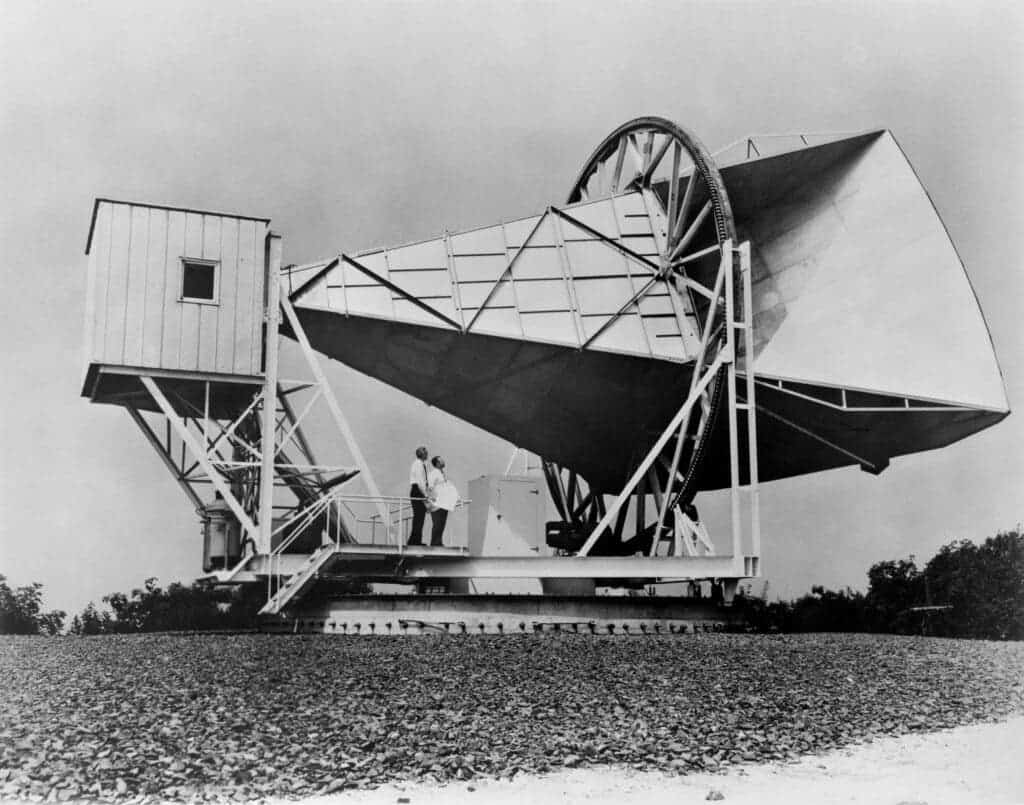
In 1964, Arno Penzias and Robert Wilson had a problem and they had no idea it was connected to the radiation field that would come to be known as the CMB. The duo was working with a 50-foot long sensitive radio horn antenna at the Bell Labs Crawford Hill Laboratory outside Holmdel, New Jersey.
Penzias and Wilson were attempting to investigate the halo that surrounds our galaxy, the Milky Way. Their investigation was being persecuted by a persistent hiss while operating the antenna that appeared to come from all areas of the sky.
The two scientists would go through a process of elimination attempting to rid themselves of the noise. Possible culprits for the hiss included the activity of New York City, the after-effects of a nuclear bomb test that had been conducted over the Pacific years before, radiation from the Van Allen Belts, or defects with the instrument itself.
“I had a lot of experience fixing practical problems in radio telescopes,” Wilson told Smithsonian Magazine in 2014. “We looked for anything in the instrument or in the environment that might be causing the excess antenna noise.
“Among things, we searched for radiation from the walls of the antenna, especially the throat, which is the small end of the horn. We constructed a whole new throat section and then tested the instrument with it.”
Eventually, the duo began to suspect that the culprit for the hiss was ‘white dielectric material’ left behind by pigeons that had taken up residence in the bowels of the antenna. ‘White dielectric material’ in case you were wondering is a very scientific term for pigeon poop.
The birds were captured, their droppings removed but still hiss persisted.
Completely unknown to Penzias and Wilson this hiss–a thorn in their side they were desperate to be rid of–would have been a dream result for a team of scientists just 40 miles away at Princeton University.
The Princeton crew led by Robert Dicke were part way through building their own horn antenna with the explicit purpose of discovering the CMB. When Penzias learned of this other team and their ambitions one of the most infamous telephone calls in the history of science took place.
Penzias called Dicke as he was eating a brown bag lunch with the rest of his team. The Princeton researcher repeated key terms said by Penzias out loud, enough for his team to realize what was happening from just one side of the conversation.
If this wasn’t enough, the dreaded confirmation came when Dicke replace the telephone receiver, turned to his team, and said: “Boys, we’ve been scooped.”
Even after the conversation, Penzias and Wilson still didn’t quite realize the significance of what they had discovered. A year and a day after they measured the CMB signal the true magnitude would dawn on Wilson when he picked up a copy of the New York Times on May 21, 1965, and read the headline: “Signals Imply a ‘Big Bang’ Universe.”
In 1978 Penzias and Wilson would be awarded the Nobel Prize in Physics for their discovery of the CMB. The Steady State model of the Universe was all but dead and Big Bang cosmology ruled the roost. But the story of the CMB doesn’t end here.
Studying the Cosmic Microwave Background Today
Discovering the CMB isn’t the end of the scientific journey of the ubiquitous radiation field, however. Baked into the CMB is the story of how the Universe has evolved, prompting the quest to map in increasing detail.
The uniformity of the CMB is one of the most convincing lines of evidence supporting the fact that the initial seconds of the Big Bang proceeded faster than the speed of light. Inflation occurring slower than this would have left large fluctuations in the CMB, and what we see in this cosmic fossil are just tiny temperature variations called ‘anisotropies.’
These tiny variations tell a vital cosmic story believed to be the result of the fluctuations in the density of early cosmic matter and energy that would give rise to galaxies and stars. That means that studying these tiny temperature fluctuations is key to understanding how the Universe evolved and the birth of cosmic structure. And doing this has relied on increasingly sophisticated technology.
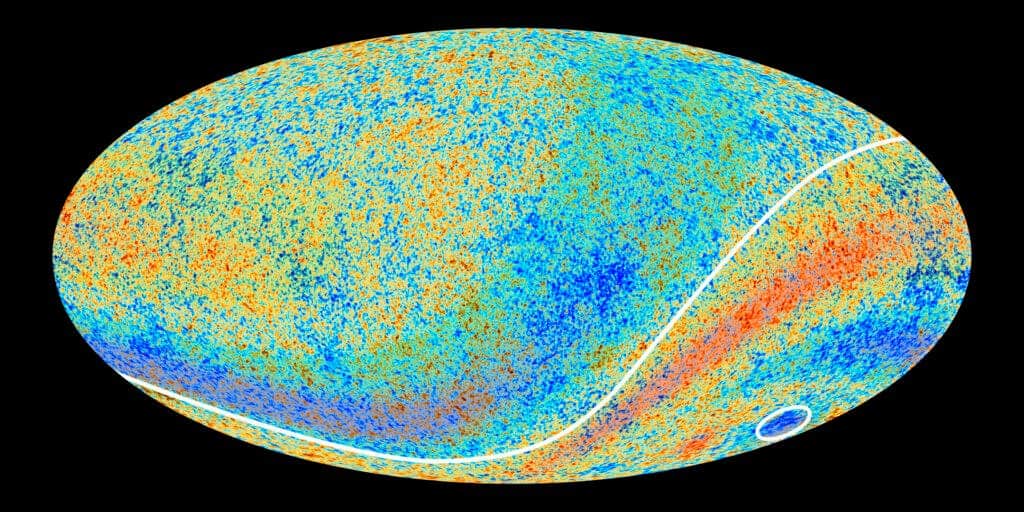
The first space mission specifically designed to investigate the CMB was the Cosmic Background Explorer (COBE). Launched by NASA in 1989, COBE found the CBE has a spectrum that conforms almost precisely to a ‘black body’ of pure thermal radiation at 2.73 K.
COBE also found tiny temperature fluctuations in the CMB across the sky on a scale of around 1 part in 100,000. The research would land John Mather and George Smoot the 2006 Nobel Prize in Physics.
These tiny fluctuations were then studied in even greater detail by NASA’s second generation space mission the Wilkinson Microwave Anisotropy Probe (WMAP) which launched in 2001.
The WMAP results have helped to determine what has become the standard model of cosmology and the course of cosmic evolution by establishing the proportions of fundamental constituents of the Universe.
Improving on this and providing the most detailed picture of the CMB to date was the mission of the European Space Agency’s (ESA’s) Planck mission, launched in 2009.
Planck does this by studying the sky in a wider frequency range, in more bands, and at a higher sensitivity than WMAP. This allows it to more accurately separate all of the components of the submillimetre and microwave wavelength sky, including many foreground sources such as the emission from our own Milky Way Galaxy.
The greater sensitivity of Planck and the revelation of the anisotropies in the CMB are both proving the Big Bang model beyond a shadow of a doubt, and supplying cosmologists with enticing hints of deviations from the standard model of cosmology.
Not bad for a cosmic fossil over 13 billion years old and once confused for pigeon poop!