Neutron stars are one of the most amazing things we know of in the universe. A teaspoon of neutron star material would weigh around a billion tons, making them some of the densest objects in the universe, second only to black holes. Aside from being extremely dense, they can emit bizarre pulses, and sometimes, they form in binary systems — where things can get even wilder.
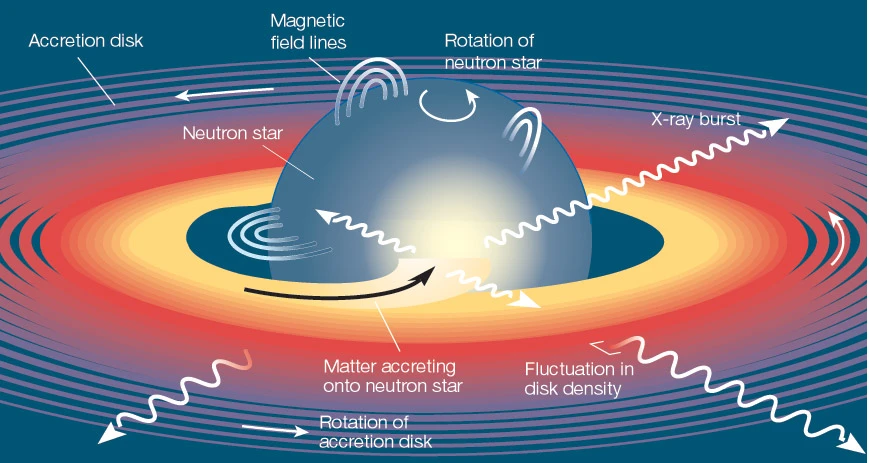
In 1969, Jocelyn Bell detected the first neutron star. She was a PhD student at Cambridge University and detected a very powerful and extremely regular radio pulse (which was later named a pulsar). It was so eerily regular that the signal’s first nickname was LGM-1, “Little Green Men 1”.
The people who later got involved in the study didn’t really believe it was another civilization and set out to find the signal’s underlying cause. They found it to be a neutron star, the collapsed core of a massive supergiant star that wasn’t quite massive enough to turn into a black hole.
Today, we can detect neutron stars by looking at their signals with X-ray detectors, and we’ve learned quite a lot about them.
Boom
Neutron stars are born when a star with 8 to 20 solar masses runs out of fuel. The star undergoes a number of nuclear fusion reactions which leave behind a layered onion shape, including a core made of iron. The iron core is the key to how the star will develop; if the core’s mass is above a limit (called the Chandrasekhar limit), the star will collapse into a neutron star or black hole. Stars with masses lower than this limit will remain stable as white dwarfs.
The formation of a neutron star can happen at dazzling speed. A supernova occurs within 0.1 seconds and what is left behind from the primary star is just its core, which is now made out of neutrons. The explosion releases neutrinos which are antisocial particles that don’t interact with almost any other particles.
In 1987, a supernova exploded and we detected neutrinos from outside of the solar system for the first time in the Kamiokande detector.
The neutron star left from the colossal boom has nearly 1.5 solar masses, but its radius is just around 10 kilometres, making it the densest star in the universe we know of – one tablespoon of a neutron star contains billions of kilograms. Mostly made of neutrons, of course, but also with some protons and electrons here and there, without the extra particles it wouldn’t be stable enough, the neutrons could decay into protons and electrons.
Interestingly though, neutron stars don’t collapse on themselves despite their massive gravitational attraction and are generally stable.
This happens because neutrons are essentially fermions, subatomic particles that respect their own personal space; you could say they practice subatomic social distancing.
In more scientific terms, fermions obey the Pauli exclusion principle: “you can’t have identical fermions in the same quantum state”. This means the identical neutrons can’t occupy the same space, thus, the pressure from ‘trying to avoid other neutrons’ personal space’ competes against gravity and the neutron star keeps itself stable for a long time. This type of matter is often referred to as “degenerate matter” — a highly dense state of fermionic matter in which the Pauli exclusion principle exerts enough pressure (in addition to, or in lieu of thermal pressure) so that the neutron star doesn’t collapse.
Classifying neutrons stars
There are several ways to classify neutron stars, but commonly, there are three types of neutron stars.
The easiest ones to find are the pulsars. Pulsars are highly magnetized rotating neutron stars that emit beams of electromagnetic radiation from their magnetic poles. They have a highly periodic pulse, which can repeat a cycle within milliseconds or over several seconds. The rotation and the beam don’t necessarily need to be aligned, that’s why most images illustrating pulsars show the tilted version of the cycles.
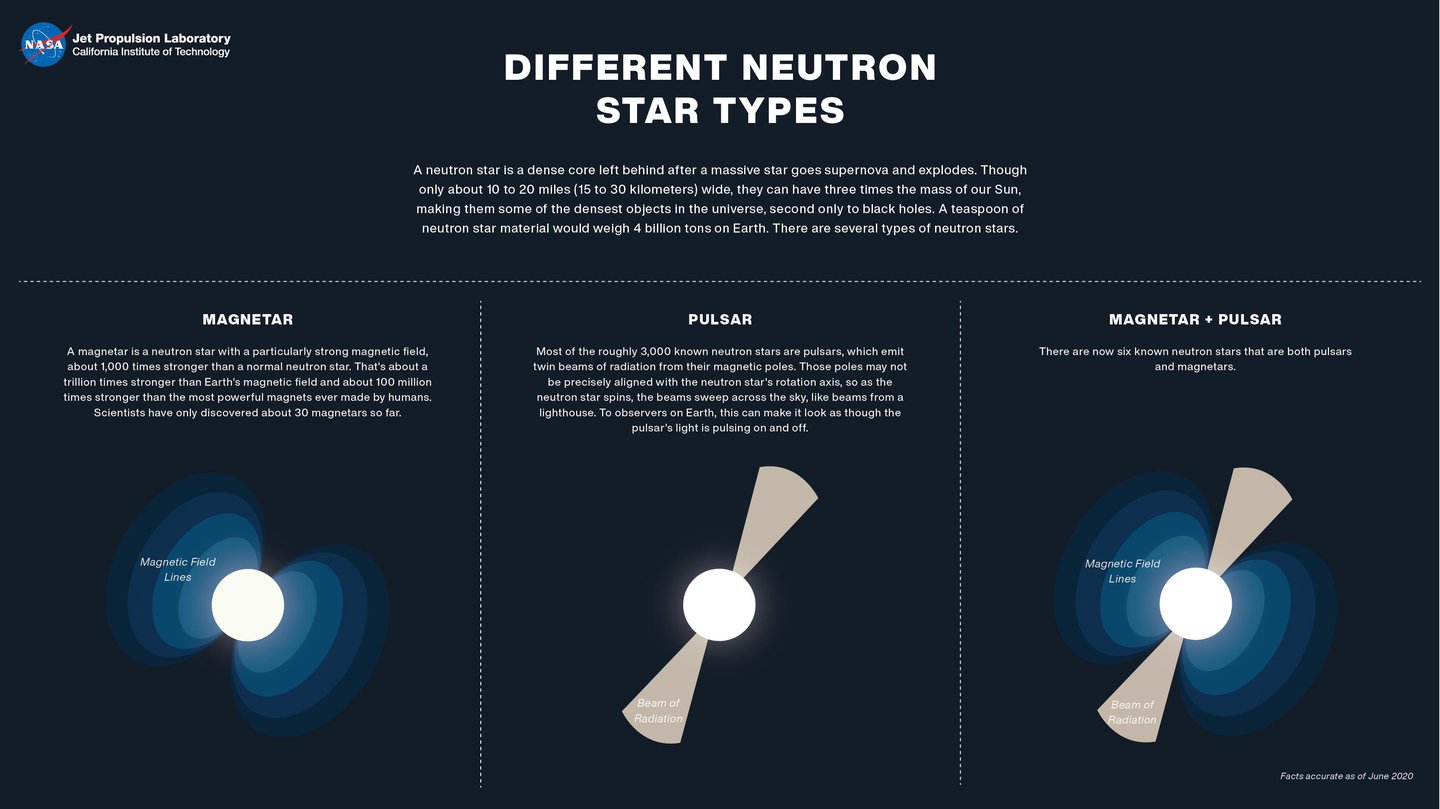
Pulsars are very serious about their timing — so much so that astronomers sometimes use them as celestial timekeepers. The timing from their pulses can be used to precisely find objects, just like how sailors of yore used stars to guide themselves at sea. The Voyager spacecraft has a message to any civilization to find Earth. How did they map it? With radio pulsars. The positions of galactic pulsars are placed on a scale in the map with their number of rotations per cycle dashed along the lines.
Another neutron star is the magnetar, and here, things really get extreme. Pulsars are already extreme objects with titanic magnetic fields, but the magnetars have fields 1000 stronger than even that of a pulsar. To get an idea of how big a field we’re talking about, the Large Hadron Collider has magnets to help accelerate particles, and its magnetic field is around 8.3 Tesla. Magnetar’s magnetic field is 10,000,000 billion Tesla. They can also cause giant flares, and release energy 100,000,000 billion times stronger than a solar flare.
Binaries
Neutron stars can orbit a companion, sometimes a white dwarf, sometimes a main-sequence star, or even another neutron star. Things get weird when they start to merge.
Credits: NASA’s Goddard Space Flight Center/CI Lab
Download this video in HD formats from NASA Goddard’s Scientific Visualization Studio
On 2017 August 17, both the Virgo and Laser Interferometer Gravitational-Wave Observatory (LIGO) teams detected two neutron stars merging gravitational waves in another galaxy called the GW170817. Because the merging process was so catastrophic, the event emitted gamma rays detected by the Fermi Gamma-ray Space Telescope. There were also visual signals from that event and other important measurements, multi-messenger observations, something widely anticipated as the future of astrophysics.
Superfluid
Remember the Pauli exclusion principle? Well, we’re not done with it just yet. There is a phase of matter which is like a fluid but not really, and it works in the same way as the superconductors.
NICER will confront nuclear physics theory with unique measurements, exploring the exotic states of matter within neutron stars through rotation-resolved X-ray spectroscopy. Credits: NASA/Nicer.
When you try to join particles with the same charge they repel each other. But at a very specific temperature and in the case of neutron stars, density, they can get along and ignore the social distancing. Superconductors have a weird mechanism to form interactions between electrons, the Cooper pairs. These interactions make the superconductor have zero resistivity, for a superfluid, it means zero viscosity, a property in fluids that make them flow slowly.
Neutrons aren’t supposed to form pairs either, even though they aren’t charged. However, in that extreme environment, they manage to form this superconducting phase that is actually called a superfluid state. It happens inside the neutron star’s inner crust and outer core.
The Cooper pairs made of neutrons make a superfluid state possible in a neutron star. It may sound weird to call it fluid in such a dense object, but if you think about it is not a problem, everything is dense, and the core is denser, a less dense region compared to a super dense one can be called a fluid.
The evidence for that is the result of the pairing. The relationships between neutrons aren’t stable so they break up and emit neutrinos in response, this neutrino release makes the star cool down. Two groups independently detected the cooling mechanism from the neutron star inside the Cassiopeia A. The 10-year observation shows that the star cooled 4%, the best explanation is that it agrees with the superfluid theory.

That was just a few of the quirky characteristics of neutron stars, they can get weirder than that. Exotic stuff probably happens in their inner cores, explained with more quantum mechanics which could make ‘Rick and Morty’ seem old hat.