Energy — we need it to stay alive. But what exactly is it, and what ‘flavors’ does it come in? Let’s find out.

Image via Pixabay.
Just as there are many different ways to do work, there are also many types of energy. As a general guideline, we split it up into two major types and several subtypes. Physicists measure energy in joules, although a more familiar unit of measure might be the calorie. With that crash introduction, let’s take a look at:
Potential and Kinetic energy
The two slices of the energy pie (in how we interpret it, at least) are kinetic and potential energy. Every type of energy we’ll be discussing today is a particular form of either of these two. Kinetic energy is energy actively performing work right now (such as moving an object or heating it up) while potential energy is what is currently ‘in storage’, which can be released if the right circumstances are met.
Energy cannot be created or destroyed, but it can be transformed. If you, for example, lift an apple over your head, you’re transforming kinetic (motion) energy into potential energy (the apple wants to go down and will do so if you let it go). As it falls, all the potential energy you’ve stored inside the fruit is turned back into kinetic energy. Alternatively, a battery holds chemical (potential) energy. It can be turned into electrical energy, then into light and heat in your smartphone (both types of kinetic energy).
So let’s start by looking at one of the most fascinating, in my eyes, types of energy:
Thermal energy
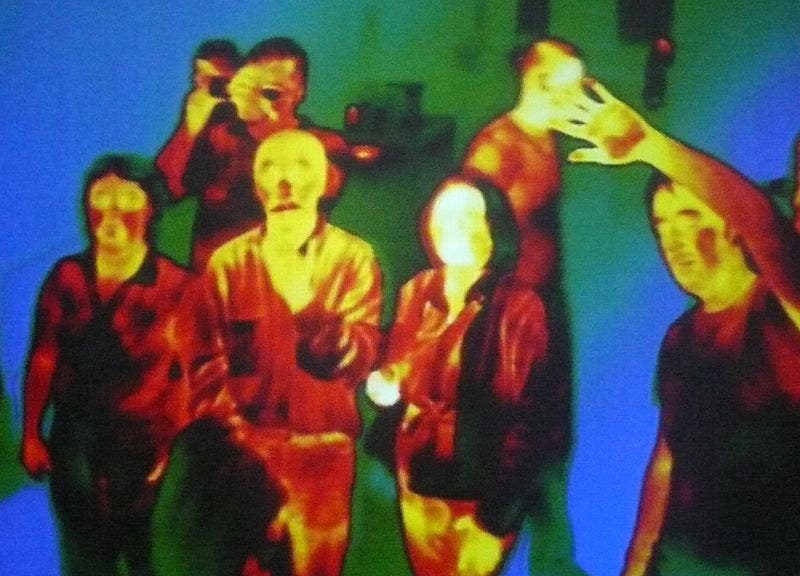
Image credits Nevit Dilmen / Wikimedia.
As a rule of thumb, all energy bends the knee to the principle of energy transformation except thermal energy. Please note at this time that what we perceive as heat isn’t thermal energy per se, but the transfer of thermal energy. Something that feels warm to the touch has more thermal energy than you — and you’re receiving it. Something that feels cold draws energy from you. The energy flow is what you perceive as ‘hot’ or ‘cold’.
Temperature, then, is how densely-packed thermal energy is in an object.
Thermal energy itself is the disorderly movement of particles inside an object. It is the sum of the kinetic and potential energy of molecules moving, rotating, or vibrating in a random manner. Thermal energy is randomly distributed among these particles or atoms, and as such is a measure of entropy — a physical’s system lack of order or predictability. The second law of thermodynamics says that the entropy of an isolated system never decreases. In plain ‘ol English, this second law basically says that you can’t take a hot object (high entropy state) and cool it down (low entropy state) without draining that energy somewhere else.
In a roundabout way, that also means thermal energy can’t be transformed, only transferred. There’s nothing wrong with thermal energy, but it is so disorderly that we can’t effectively channel it to transform it. Thermal energy also wants to even out as much as possible over as wide a volume as possible (ideally, across the whole Universe, in its book). This, alongside its workless nature, is why thermal energy is often seen as a ‘residual’ type of energy that all other energy degrades into.
The interplay between thermal energy and physical work are enshrined in the first law of thermodynamics. This law also shows us how heat, i.e. thermal energy imbalances, can be used to perform work. In short, it says that the energy state of a closed system is the difference between changes in heat (gains in energy) and work performed (energy expenditure).
Thermal energy itself can’t perform work, but an imbalance and subsequent transfer of thermal energy can. A hot oven is more energetic than a cool oven, but neither move by themselves. The fires bellowing in a steam locomotive’s furnaces don’t directly drive the thing forward. They heat up water, however, which then expands into steam, and this (heat-induced) change in state and volume is converted into motion. If you want to get all physical about it, the motions of individual water particles can get so hectic that it turns to steam; the motions of these steam molecules then get transferred (via impact) to the various pistons they drive in the engine, effectively converting thermal energy flow into motion.
So, to sum it up, thermal energy is the hipster of energies. By itself, it cannot be converted into other types of energies. Only differences in thermal energy can be transformed/used to perform work. The efficiency of such processes will never be 100% — you will never be able to recover all the energy in heat.
Mechanical energy
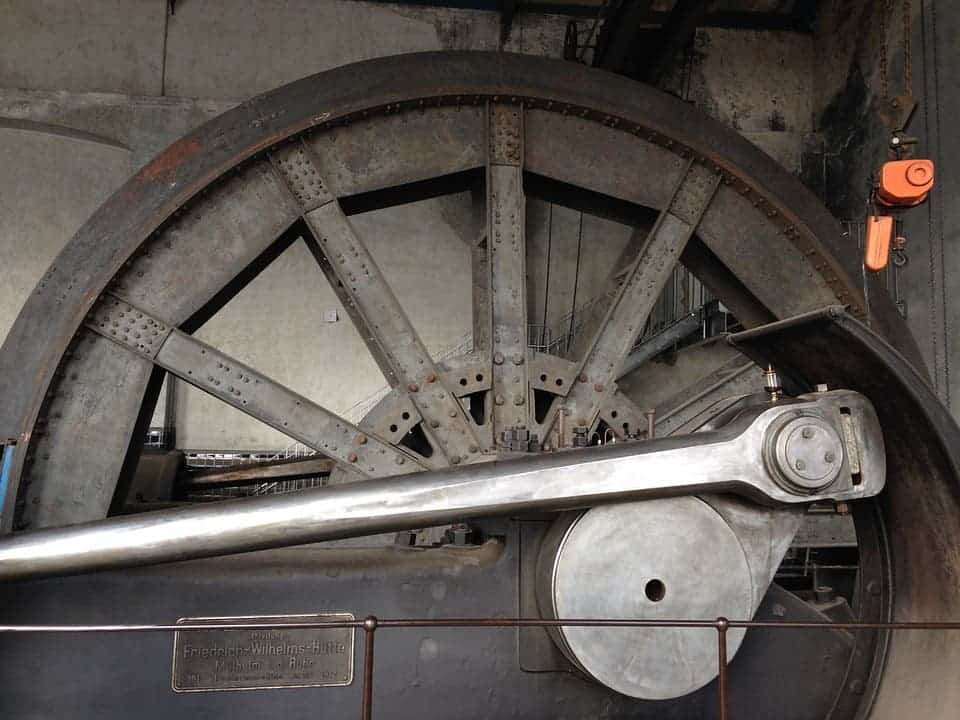
Image via Pixabay.
Mechanical energy is the total potential and kinetic energy resulting from the movement, or current location, of physical objects.
Kinetic mechanical energy characterizes physical bodies in motion and is half the product between its mass and the square of its velocity. The heavier something is, and the faster it moves, the harder it is to stop (i.e. the more kinetic energy it has). Potential mechanical energy depends on the body’s position relative to other bodies.
Potential mechanical energy is often associated with forces that apply work against the field of a conservative force. Conservative forces are forces independent of the path of motion, such as gravity or electrostatic interactions between particles. The easiest way to illustrate potential mechanical energy is by imagining you’re carrying a bucket of water up a flight of stairs. If you then dump the water, it will flow down to the ground. You stored potential energy in the water by acting against the gravitational field (i.e. you lifted the water). When you released it from the bucket, that water expended its potential energy as kinetic energy under the action of gravity.
An interesting property of mechanical energy is that in an isolated, ideal system, it is constant. In real systems, however, non-conservative forces (such as friction or air drag) will eventually sap mechanical energy, turning it into heat.
Chemical energy
Do you know what has a lot of chemical energy? Chocolate. But, if you want something with a lot of chemical energy, you need dynamite.

Image via Wikipedia.
Chemical energy is potential energy stored inside a substance’s chemical bonds. Our bodies break open bonds during cellular respiration to obtain this type of energy. Chemical energy is also released when we blow up a stick of dynamite, when feeding wood into a fireplace, when pressing the gas pedal, and as the battery in your smartphone generates electricity.
If a substance can react with another to undergo a transformation through a chemical reaction, it has chemical energy. That energy is equal to the difference between the energy content of the products and the reactants (if the temperature remains constant). It doesn’t much matter what, exactly, that change is — it can be a change in how a molecule’s atoms are arranged; it can involve the breakdown and creation of new products. As long as a chemical change takes place, it will either generate or absorb energy.
Combustion, that merry thing keeping the world going, is a superb example of chemical energy being released. Fire is what happens when oxygen molecules bind to various compounds, releasing the energy in their bonds.
Electrical energy
This type of energy is the result of the flow of electric charge through a conductor due to electrical attraction or repulsion between charged particles. Electrical energy can be potential (static electricity) or kinetic (when the charges are in motion, i.e. electrical current).
It is generated from differences in electrical potential between two or more objects in a given system. It can also be generated by kinetic force, though the movement of a copper wire loop or disk around the poles of a magnet. Generally speaking, this works because the electrons in the copper wire are free to move about as they please.
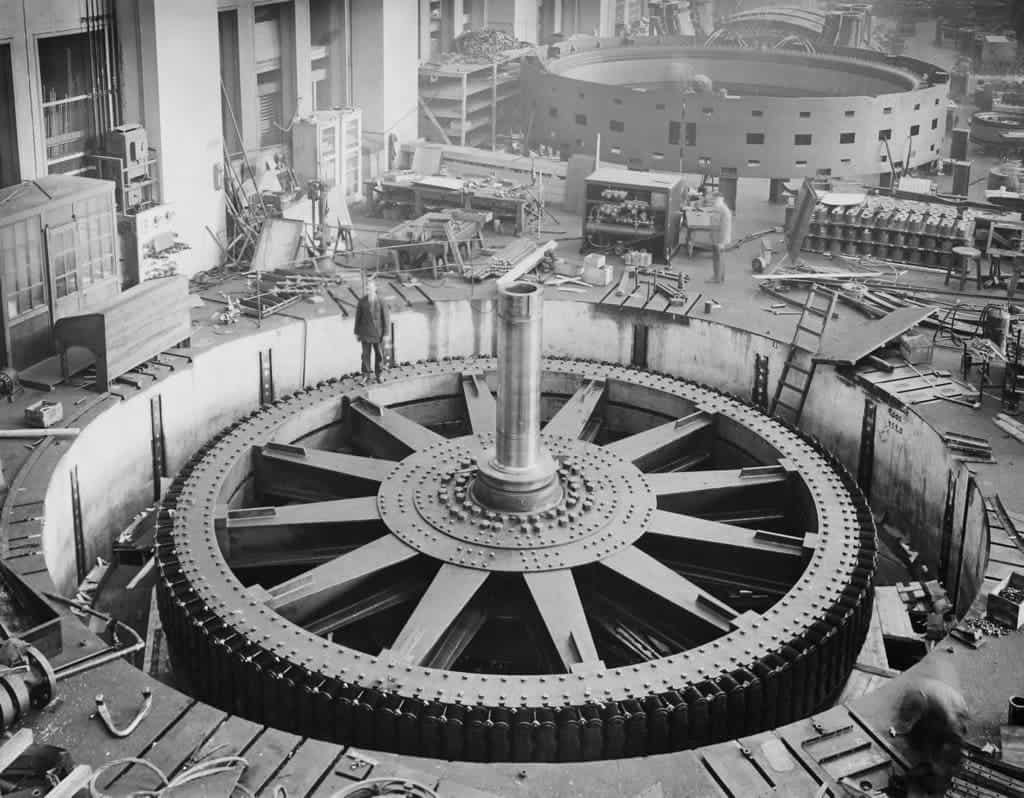
Image credits Tekniska museet.
Each electron is negatively charged, so it will be attracted to positively-charged particles and pushed away by other negatively-charged particles. You can also see this as the electron attracting certain particles while repulsing others — in other words, each charged particle has a tiny electric field around it that can exert a force on other particles, causing them to move (force over distance is physical work). Generators function by supplying force to move these charged particles around, causing them to move other charged particles, in turn, generating electricity.
A moving charged particle will always generate a magnetic field. A moving magnetic field always induces an electric current in a conductor. That’s why these two are usually clumped together under the banner of ‘electromagnetism‘.
Nuclear energy
Nuclear energy is released (or absorbed, mind you) whenever a nuclear reaction, or radioactive decay, occurs. It is the product of differences in the nuclear binding energy of the first and final state of these transmissions. The nuclear binding energy of an atom is defined as the minimum energy needed to break it apart.
In essence, all atoms are made up of particles and the forces holding these particles together. Different types of atoms need different amounts of force to keep them together. When an atom undergoes change, or when it is split, this energy is released. Nuclear energy, therefore, is potential energy.
In any exothermic (net-energy-positive) nuclear process, nuclear mass might ultimately be converted to thermal energy, and given off as heat. Fission and fusion are the best-known nuclear transformations that release energy. The Sun and all other stars are directly powered by nuclear fusion.
Depending on how technical you want to go with the classifications, you could draw up other types of energy. Elastic energy describes how stretchy things revert to their shape when you let go, for example. However, I tried my hardest to give you the overarching ‘flavors’ of energy, ones that can reasonably fit all other subtypes (elastic energy is a form of mechanical energy). But, if you feel I left something interesting out, perform some physical work on your keyboard and let me know in the comments below!