No other year in living memory has been as heavily influenced by a virus as 2020. But what exactly are viruses, what makes them tick, what about them made us all put our lives on hold?
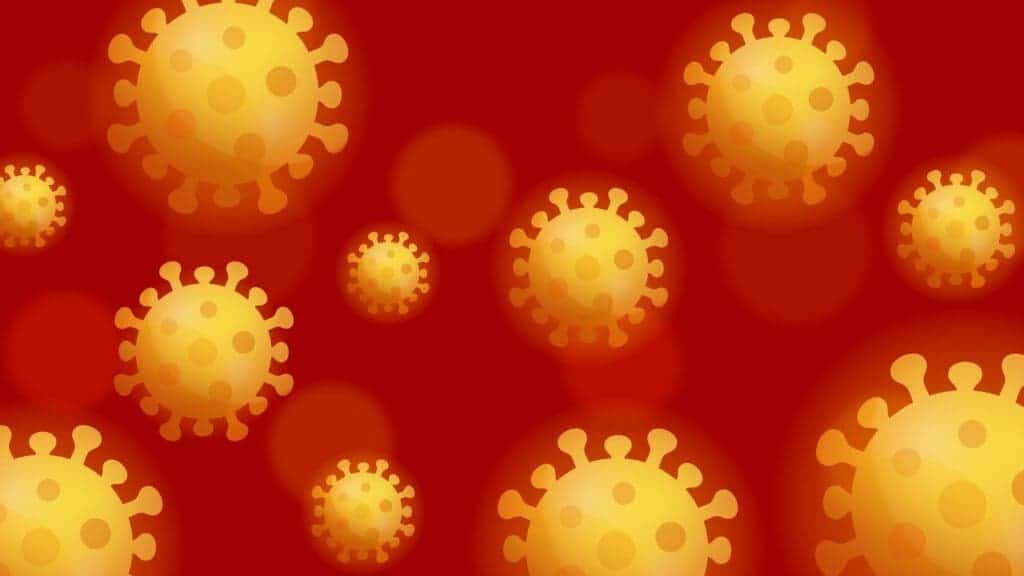
The short of it is that viruses are biological machines, supremely well-adapted to a single survival strategy. And this strategy is quite simple — viruses find living cells, infect them, and hijack their biochemical machinery to reproduce. They’ve done away with (or perhaps never developed in the first place ) anything that doesn’t directly help them perform that task, all the way down to the most fundamental traits of living organisms: viruses aren’t really alive, but they’re not not-alive either.
Their simplicity works to make viruses easy to ‘build’ (so they’re plentiful) and hard to detect and destroy. In fact, we still have very few reliable medicines against viruses (they’re known as ‘antiviral’ compounds), and they often only work on particular kinds or lineages of viruses. We’ve managed to put a man on the moon and put stars inside a bomb, but for all our achievements, humanity’s best defense against these pathogens is still our own bodies and immune systems. This becomes a bit scary when you consider that viruses very definitely outnumber any and all living things on the planet.
But I’m getting ahead of myself. Let’s start from the beginning:
General viral structure
Viruses are acellular. This means they are not made from cells nor do they have a cellular structure. This also means that all those fancy components you may or may not have learned about in cellular bio 101 — organelles, plasma membranes, ribosomes, etc — have nothing to do with a virus. They’re also exceedingly tiny, typically around 20–300 nanometers in diameter, though a few are larger. To put things into perspective, if a bacterium was the size of a soccer field, a virus would be around the size of three soccer balls put side-by-side. An animal cell would be the town around it.
One such pathogen (an individual, fully-assembled virus is referred to as a ‘virion’) is about as simple a biological machine as you can make and still have it work. They include a core that houses their nucleic acid (genetic material), an outer coat of proteins or ‘capsid’, and that’s pretty much it. That’s all you need to make a working virion. However, some fancier models can have additional features, such as an outer membrane shamelessly stolen off a host cell, different proteins (or glycoproteins) that can help them infect targets, or other structural elements. Capsids are constructed from proteins known as capsomeres. Them, alongside any membrane viruses have, typically tend to be peppered with glycoproteins that serve as binding or access keys into certain cells.
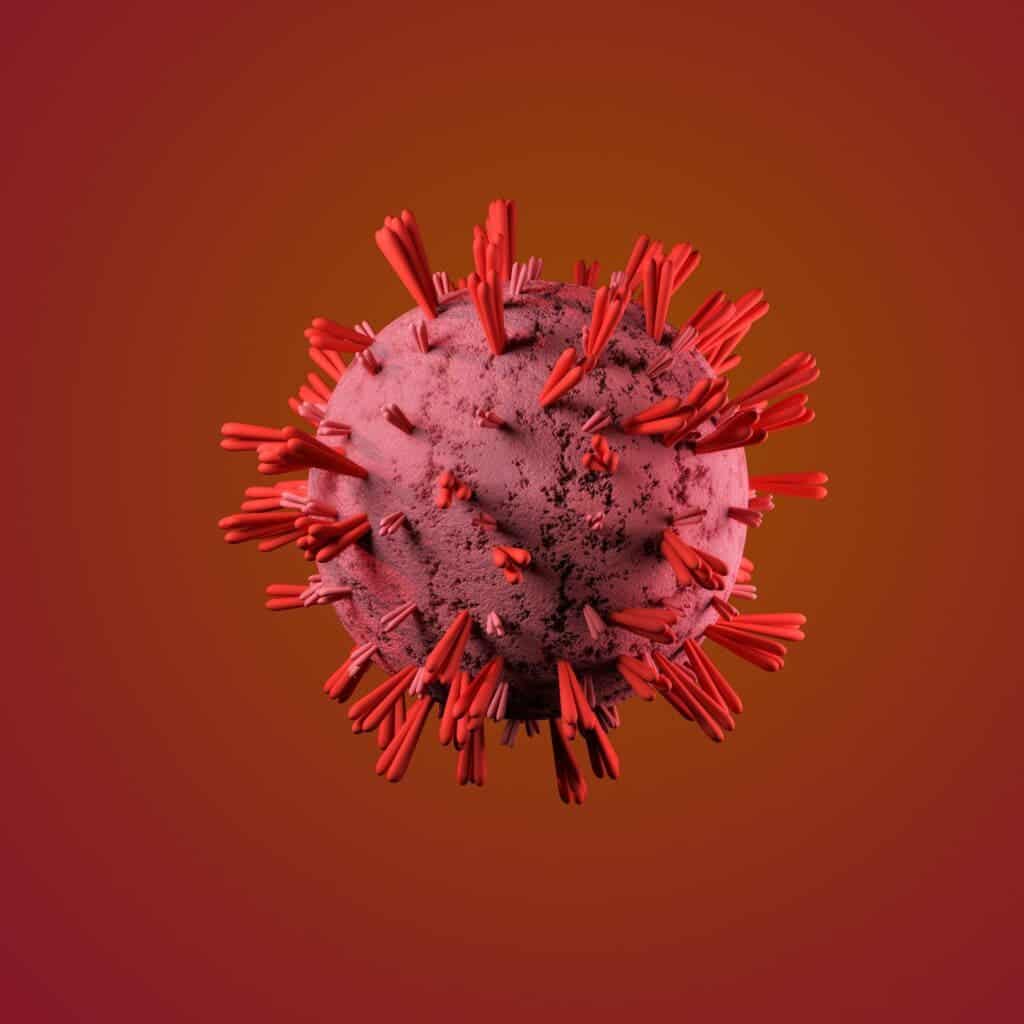
By and large, viruses are classified into one of four groups based on their structure: filamentous, isometric (or icosahedral), enveloped, and head and tail. We’ll be getting to them in a second. One interesting characteristic of viruses is that across all strains, their complexity seems to be in no way related to the complexity of their hosts. The most complex and intricate structures we’ve seen in viruses belong to bacteriophages, pathogens that infect bacteria (which are the simplest living organisms).
What shape a virion takes, as well as the presence or absence of an envelope, has little bearing on what species it can infect and what the symptoms would be, but they’re still very useful classification criteria because they’re relatively easy to check.
Viral morphology
The shape and size of viruses tends to be consistent among different lineages, and quite distinctive for each.
Filamentous viruses have long, cylindrical bodies; plant viruses often employ this shape, including the TMV (tobacco mosaic virus). Icosahedral or isometric viruses look pretty much like spheres, or spheres with flattened faces. They get their name from the icosahedron, a polygon with 20 faces (like the dice you use in Dungeons and Dragons), although they don’t necessarily have to have that exact shape. One icosahedral virus you may know personally is the rhinovirus (which causes the common cold). Enveloped viruses have a membrane that surrounds their capsid, which is produced from bits of a cell’s membrane modified with viral proteins. The HIV virus is an enveloped virus, as most animal viruses tend to be. Finally we have head and tail viruses, which have a ‘head’ similar to icosahedral viruses and a ‘tail’ that resembles filamentous ones — they often infect bacteria.
Filamentous viruses are also known as ‘helical’, as their capsomers are arranged around a coil of genetic material, forming a helix. Them, alongside icosahedral viruses are sometimes called ‘simple’ viruses, while head and tail ones (or other shapes) are known as ‘complex’ viruses.
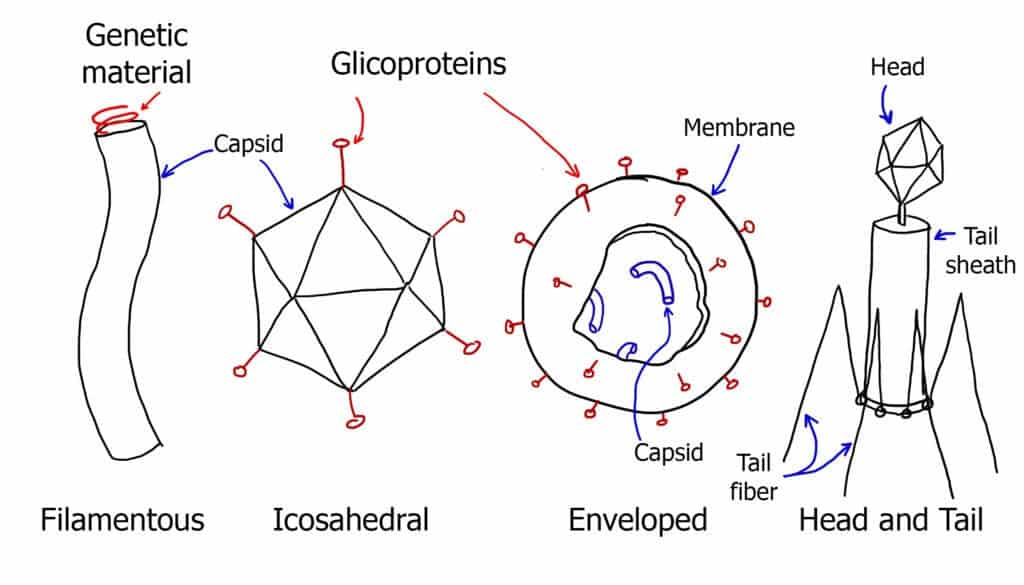
The presence of a membrane can help facilitate infection and provide protection against the host’s immune system (as it’s made from pilfered parts of cells). Enveloped viruses tend to rely completely on their membrane for infection. Its glycoproteins exploit cells’ natural pathways through the membrane to allow infections. They act as ‘keys’ to the protein ‘locks’ that are typically employed to allow nutrients or other elements through the lipid layers of the membrane. But through this, they become vulnerable to inactivation by compounds that interact with fats, such as soap. This is the case for the coronavirus, for example, which is why handwashing is so effective against it.
All of this is very swell, to be sure, but why are viruses so interested in getting inside cells? So glad you asked — here’s why:
The viral life cycle
The central idea to keep in mind here is that viruses aren’t technically alive. They have some of the trappings of living things — genetic material, they’re made of organic matter — but they also lack most essential elements of life, most notably the ability to reproduce by themselves. But that’s all fine and dandy, as far as viruses are concerned, because everyone else can do it for them.
Think of viruses as weaponized USB sticks. For the most part, they’re inert. Viruses have no metabolism, they don’t expend energy, they don’t move on purpose and they don’t chase their prey. They just float around, and every infection begins with a random encounter between a virus and a host. Once they make contact with an appropriate cell, a six-step process unfolds: attachment, penetration, uncoating, replication, assembly, and release.
Attachment and penetration are pretty self-explanatory. They involve the virion coming into contact with and attaching to the host cell, and the subsequent penetration through its membrane. Attachment is governed by the type of binding proteins on the capsid and the transfer proteins on the cell wall — if they’re compatible, the process can unfold. Penetration involves the transfer of viral genetic material through the membrane, which leaves the capsid outside the cell; in this step, the virion basically injects its genetic data into the host cell. Note that some enveloped viruses use other tricks to get inside the cell, most notably by fusing their membranes with that of the cell or tricking it into eating the virus. Once inside, the capsid degrades and the genetic material is released, representing the Uncoating phase.

In regards to this genetic data, first know that it can be either DNA or RNA (some virions that carry RNA are known as ‘retroviruses’). Viruses can carry single or double strands of DNA (‘ssDNA’ or ‘dsDNA’ viruses respectively) or RNA (‘ssRNA’ or ‘dsRNA’). Single strands can be either sense or antisense. Sense strands are those used actively as instructions to create proteins (messenger RNA, or ‘mRNA’), while antisense RNA are their mirror complementaries and serve as a template to create strands of mRNA.
Now, the moment we’ve all been waiting for: what does this genetic material encode? Well, the complete information on how to build the virus, naturally! Once the viral material enters the cell, it will hijack its ‘code’ to make it produce more viral genetic material, capsid elements, and anything else that is needed to Replicate the original virus. DNA viruses typically use a cell’s biochemical machinery to create more DNA (for the new viruses) that is then transcribed into mRNA, and this mRNA is used to start protein synthesis. RNA viruses use their genetic code as a direct template for more RNA (for the new viruses) and mRNA that is consumed in protein synthesis. Retroviruses such as HIV contain RNA that must first be copy-pasted into the host’s DNA — but they also have the right protein, ‘reverse transcriptase’, for the job.
If a cell lacks the know-how required to build these various elements, the viral genome instructs it on what needs to be done. This is best exemplified by retroviruses. Reverse transcription or retrotranscription involves turning a strand of RNA into a double-strand of DNA which is then inserted into the host genome; in very broad lines, it’s reverse-engineering, like creating a full blueprint on how to build a car by just looking at the car. Very nifty. Bacteria and cells do use reverse transcription, but typically for what could be considered data maintenance work. It’s unclear whether this is a natural ability of all cells or if it was inherited from ancient viruses that grafted the needed genes into their hosts (which goes to show that viruses can be a driver of evolution).
The bits and pieces that the cell creates will spontaneously self-Assemble into new virions inside the cytoplasm. Finally, they Release (or ‘egress’) out of the cell. Exactly how this takes place varies from strain to strain. Some viruses (especially enveloped ones, including HIV) gradually exit the cell through budding — a process through which they also gain their membrane covering — which keeps the host cell alive. Most commonly, however, virions are released when the cell is so full of viruses that it bursts open (and dies in the process).
Lytic vs Lysogenic
Now, viruses may seem evil, but they’re not out to kill you. In fact, they will occasionally put in the effort to not hurt their host, especially during times when prey cells are rare and harder to find.
The process of a cell ripping apart, the breaking down of its membrane, is known as ‘lysis’. Under normal circumstances, virions follow the lytic cycle, which is the one described above that ends in the death of the cell. Such an event sees several hundred virions released from the dying cell, around 100 to 200 individual particles, as a rule of thumb.
The lysogenic cycle is a bit more covert — it produces something known as a ‘temperate’ or ‘non-virulent’ infection. Through the lysogenic cycle, a virion lies dormant and hidden inside the host cell genome, waiting for the right time to strike. During this time, it uses inhibitor genes so that the host cell doesn’t read the viral information, leaving it free to hang around unimpeded. The cell also profits by gaining immunity from reinfection with the same virus.
But when the cell experiences some kind of stressor (such as exposure to UV light or chemical agents) that weaken these inhibitors, its automatic DNA-repair systems detect the intruder, activate, and cut it out of the genome. After this point, the viral genetic material activates and the steps of replication, assembly, and release resumes as per normal conditions, and the infection spreads.
Why are viruses a thing?
We don’t really know. They’re too simple for us to reliably extract information on their evolutionary history from them. They’re not exactly alive, but they can and do evolve and mutate when reproducing in cells. They also have an annoying habit of copy-pasting genes from and onto their hosts, which further muddies the waters.
What we do know is that they are the single most successful group on the planet. A paper published in the journal Nature in 2011 puts their immense scale into perspective. Although it cautions that these estimates are “mostly based on ‘back of the envelope’ calculations and should therefore be viewed as they were intended: ballpark figures aiming to inspire”, they’re still no less impressive.
“If all the 1 × 1031 viruses on earth were laid end to end, they would stretch for 100 million light years. Furthermore, there are 100 million times as many bacteria in the oceans (13 × 1028) as there are stars in the known universe. The rate of viral infection in the oceans stands at 1 × 1023 infections per second, and these infections remove 20–40% of all bacterial cells each day.”
“There are about 200 megatonnes of carbon in viruses in the ocean, which is equal to about 75 million blue whales,” explains Curtis Suttle, a Distinguished University Scholar and Professor at the University of British Columbia in another paper. “In fact, in a litre of coastal seawater there are more viruses than there are people on the planet.”
“If aliens randomly sampled Earth they would see a planet dominated by microbial life, most of which would be viruses,” Suttle adds. “On average, there are about 10 million viruses and a million bacteria per litre of seawater or freshwater. If we compare the number of viruses in the oceans to the number of stars in the universe, there are about 1023 stars in the universe [and] about 10 million-fold more viruses in the ocean.”
These numbers showcase why humanity can never truly hope to ‘defeat’ viruses — it hasn’t ever been an option. But one of the best, and perhaps most chilling ways to illustrate this is the legacy viruses have left in us.
Viruses have, to the fullest extent of the word, become a part of us. It’s estimated that around 8% of the genome of modern humans is viral, meaning it has been passed from a virus into a cell, down through the generations, and we still carry that around. By contrast, only between 1% and 2% of our genome was inherited from the Neanderthals.
We are more ‘virus’ than we are our closest relatives.