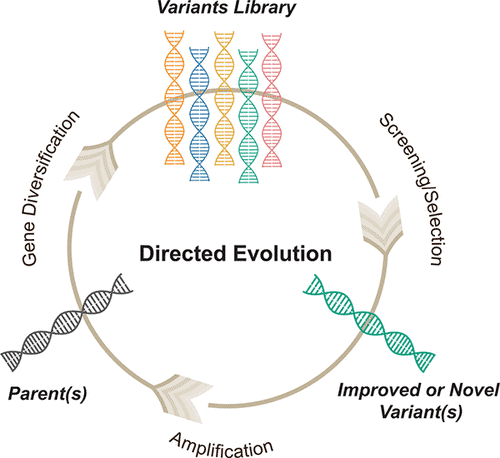
Directed evolution is a method used in biochemistry for protein and enzyme engineering, explained Frances Arnold at the 2023 Lindau Nobel Laureate Meeting. Arnold is one of the pioneers of the field — she was awarded a Nobel Prize in 2018 for the use of directed evolution to engineer enzymes.
“I wanted to be an engineer in the biological world and I fell in love with enzymes because they can do chemistry better than human chemists,” Arnold said in a lecture describing the approach.
In essence, directed evolution mimics the process of natural selection to steer the development of proteins or organisms toward a specific set of characteristics or functions. But instead of letting nature do the selection, human chemists do it. The process generally works like this:
- Mutation. First, you introduce a genetic variation into a population of proteins or enzymes. This mutation can be random, but it’s useful to mutate the genes that code for the trait of interest.
- Selection: Next, these variations are analyzed and the most promising ones are isolated. Specifically, those that exhibit the desired traits or functions to a greater degree are isolated.
- Amplification: The selected proteins or organisms are then reproduced, creating a new population that, on average, performs the desired function better than the initial population.
- Repeating: The cycle of mutation, selection, and amplification is then repeated multiple times to continuously improve the performance of the population. This is done until the desired result is obtained.

Evolution on speed dial
Designing molecules and enzymes that do what you want them to do is not an easy task.
“How do you create enzymes that do chemistry you cannot find? How do you encode that? I thought about this for years and I was getting very annoyed. Then I realized I was thinking about it in the wrong way,” Arnold explained in the lecture. The meeting, where Nobel Laureates meet up to interact with some of the world’s most talented young researchers, was the perfect place to discuss this approach.
Normally, you’d need to understand the exact structure that you want to create, as well as the underlying molecular mechanisms. But the beauty of directed evolution is that it doesn’t require a detailed understanding of the underlying molecular mechanisms. Instead, directed evolution relies on the powerful, iterative process of genetic variation and natural selection, guided by human-defined selection criteria. It’s essentially taking advantage of the diversity and evolution that exists in nature, explains Arnold.
“How do enzymes create new chemistry? It comes out of diversity. Novelty is already there because there’s massive diversity in the natural world. You may not know where it is, but nature will find it and it will appear in natural selection,” the pioneering researcher explained.
Credits: Lindau Nobel Laureate Meetings / Frances Arnold.
Applications of directed evolution
In nature, evolution typically takes place over eons. But in the lab, humans can direct the process much faster.
“In the lab, it’s the same rules as natural evolution, but we get to set the criteria for who survives,” explained Dane Wittrup, an MIT professor of chemical and biological engineering, in a previous release.
This method has already found extensive application in various fields. Let’s say, for instance, that you want to create antibodies that bind to a specific protein found only on tumor cells. You start a batch test with millions of yeast cells engineered to express mammalian antibodies. Then, you start adding probes containing the molecule you want to target.
At first, the odds are you won’t see much.
But then you start mutating the yeast to get them to do something different. This can be done, for instance, by irradiating them to trigger mutations. Then, you take the resulting mutations and screen them, looking for the most promising results. It may not work at first, but after enough iterations, if the selection is done correctly, you will start to see results.

Directed evolution is a cycle. In the cycle, the ‘winners’ from the first rounds of selection reproduce, creating a new generation of yeast cells. These offspring, equipped with the desired skills, then undergo further mutations. In each cycle, the yeast cells get better at the task we set. It’s akin to training a group of athletes, each generation running the race faster and enduring longer.
“Evolution, the survival of the fittest, takes place over a span of thousands of years, but we can now direct proteins to evolve in hours or days,” said Jennifer Cochran, a professor of bioengineering who co-authored a recent paper on accelerating protein evolution.
“This is a practical, versatile system with broad applications that researchers will find easy to use,” said Thomas Baer, director of the Stanford Photonics Research Center, who co-authored the paper.
So developing and improving antibodies for treating various conditions is one application. But it’s far from the only one.
Health and environment and everything else
The applications for this system range from the development of enzymes for industrial processes, to new biological pathways for synthetic biology, and novel protein drugs with better therapeutic effects. Directed evolution is a tool that allows us to harness the power of evolution to solve human problems, which makes it an integral part of modern biotechnology.
For instance, you can build nanoparticles with desired properties and use them for myriad applications. Scientists have used directed evolution to create enzymes that work at lower temperatures, leading to energy-saving laundry detergents. Many of the cosmetic products we use nowadays were formulated using directed evolution.
Increasingly, scientists are looking at directed evolution for environmental applications as well.
Researchers have shown how the process can produce efficient enzymes that open the door for more environmentally friendly ways to produce drugs and other chemicals. But directed evolution can also help us engineer enzymes that get rid of unwanted pollution. For instance, such enzymes can disintegrate plastic and other unwanted pollutants.
Virtually anywhere you need to work with enzymes and proteins, directed evolution can help.
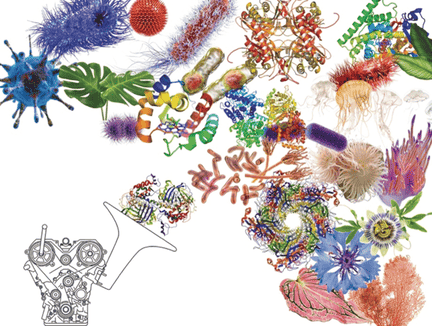
Directed evolution is used to engineer proteins with improved or novel functions. This can include enzymes with higher catalytic efficiency, stability under extreme conditions, or the ability to catalyze new reactions.
In the biotech industry, directed evolution is used to create more efficient biological catalysts for industrial processes. This can lead to more sustainable and cost-effective production methods.
Directed evolution can be used to develop new drugs or improve existing ones. For example, it can be used to modify the properties of therapeutic proteins to improve their stability, efficacy, or reduce side effects.
The approach may also be used to engineer plants or microbes with desirable traits, such as increased yield, resistance to pests or diseases, or the ability to thrive in challenging environmental conditions
In the field of bioenergy, directed evolution can be used to engineer microorganisms that produce biofuels more efficiently or to create enzymes that can break down plant material for biofuel production
Directed evolution can be used to engineer organisms that can break down environmental pollutants. This would provide a potential solution for bioremediation of contaminated sites. Also, directed evolution can be used to create enzymes that can catalyze specific chemical reactions. This would provide more environmentally friendly alternatives to traditional chemical processes.
Directed evolution can be used to create new materials with desired properties. For example, proteins can be engineered to bind to specific materials, creating the potential for bio-based materials with novel properties.
History of directed evolution
The main advantage of directed evolution lies in its fundamentally different approach. Most protein structures are so incredibly complex that predicting how one tweak will change their function is impossible. At the very least, it’s extremely challenging. “Nature does not care about your calculations,” Arnold explains.
The story starts in the 1960s, when researchers started paying more attention to RNA molecules. Sol Spiegelman, a researcher at the University of Illinois at Urbana-Champaign, showed that the principles of natural evolution also operate at the molecular level. This is now called the “Spiegelman’s Monster” experiment. The concept was then extended to protein evolution.
New methods to evolve and screen enzymes were developed in the 1990s, bringing the method to the forefront of biochemical research. The field rapidly expanded with new gene variant libraries and tools, and the Nobel Prize awarded in 2018 pushed the method even more.
Now, there are thousands and thousands of published studies on the directed evolution of enzymes and proteins, and new ones are getting published each year.
The future of directed evolution
Although the basics of the method were developed decades ago, researchers are just now starting to explore the full potential of this method. Just a few years ago, for instance, Arnold, found a way to engineer a protein to create a bond between carbon and silicon atoms. This is significant because while carbon-silicon bonds are common in the world of synthetic chemistry (used in things like plastics and electronics), they are virtually non-existent in biological systems.
Meanwhile, other researchers are looking at developing new antibiotics, using the “genetic scissors” CRISPR/Cas9 alongside directed evolution, or even deploying directed evolution on other organisms. Just imagine, the humble yeast — the organism inside a savory slice of freshly baked bread — could also be helping to clean our environment. By using directed evolution, scientists have tweaked yeast to devour harmful pollutants, or act as a sensor, or kill weeds.
It sounds like science fiction, but this is what’s happening in labs around the world today.
Machine learning algorithms are also starting to play a role in this technology. After all, when it comes to spotting patterns (say, patterns in a group of enzymes you’re mutating), AI truly shines. In fact, even language models that were not trained in biology at all were found to be useful in the process.
“The artificial designs perform much better than designs that were inspired by the evolutionary process,” said James Fraser, Ph.D., professor of bioengineering and therapeutic sciences at the UCSF School of Pharmacy, and an author of the work, which was published Jan. 26, in Nature Biotechnology.
“The language model is learning aspects of evolution, but it’s different than the normal evolutionary process,” Fraser said. “We now have the ability to tune the generation of these properties for specific effects. For example, an enzyme that’s incredibly thermostable or likes acidic environments or won’t interact with other proteins.”
As is often the case in science, advancements in one field often spill to another, accelerating progress. This is exactly what we’re seeing with directed evolution.
Future Hopes and Ethical Dilemmas
This kind of breakthrough didn’t happen overnight. Evolution is a vast, complex maze, and finding the right path requires time, patience, and a bit of luck. But there’s another maze looming on the horizon: an ethical one.
As the promise of directed evolution is immense, it also forces us to ask important questions. As we take the reins of evolution, what ethical boundaries must we set?
Picture a future where organisms are custom-built for any task we can dream of. From supercharged crops that can thrive in harsh climates, helping to solve world hunger, to genetically modified animals with enhanced abilities. How do we ensure that this power is used to lessen inequality, instead of exacerbating it? How do we use this power to make the world a better place for everyone, instead of a select, chosen few?
As we unlock the secrets of life, we must remember to tread with care. It’s up to us to ensure we use this power wisely, balancing progress with respect for the intricate web of life that surrounds us.
Ultimately, directed evolution is a testament to our scientific understanding and growth. We can learn to harvest natural processes and then use them to our advantage in ways that seem almost unbelievable. The story of directed evolution is a testament to human ingenuity and our ability to learn from and harness the power of the natural world. As we continue to explore the potential of this technology, there is no doubt that directed evolution will play a crucial role in shaping the future of science and technology.
In the words of Frances Arnold, “Nature has been conducting experiments for billions of years. We just need to learn how to listen.” As we continue to learn from and build upon nature’s wisdom, the possibilities are limitless.